57 8.5 Nitrogen and Nutrients
Nitrogen is the most abundant gas in the atmosphere, and like the other atmospheric gases it dissolves into the surface layers of the ocean. But most marine organisms cannot directly utilize dissolved nitrogen in the form in which it exists in air (N2), so it must first be converted into other nitrogenous products by marine bacteria (Figure 8.5.1). Some bacteria (cyanobacteria) take the dissolved N2 and convert it into ammonium (NH4+) through nitrogen fixation. Some of this ammonium can be used directly by phytoplankton, but the majority of it is converted by bacteria into nitrite (NO22-) or nitrate (NO3–) through the process of nitrification. Nitrate is the main nitrogenous compound utilized by primary producers in the ocean; it is a major nutrient required for photosynthesis. Note that in this context, a nutrient refers to a chemical needed to support photosynthesis and primary production. It does not refer to the nutritional needs of consumer organisms. The nitrogen taken in by phytoplankton gets passed on to consumer organisms, and then gets returned to the ocean through decomposition of wastes and organic matter as these organisms die and sink into deeper water. Finally, the ammonium, nitrate and nitrite can undergo denitrification by yet another group of bacteria and get converted back into N2, which can reenter the cycle or be exchanged with the atmosphere (Figure 8.5.1).
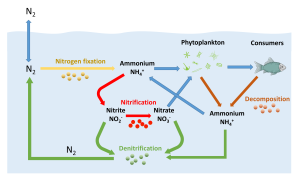
Since nitrate is one of the most important nutrients, for now we will focus only on nitrate as we discuss general nutrient patterns in the ocean. A representative nutrient (nitrate) profile is shown below (Figure 8.5.2). Since nutrients are rapidly used in biological processes, they are non-conservative, and their concentrations vary regionally and seasonally. Nutrient concentrations are low at the surface, because that is where the primary producers are located; the nutrients are rapidly consumed and they do not have the chance to accumulate. Nutrient levels increase at depth, as they are no longer being consumed by producers, and they are being regenerated through the decomposition of organic material by bacteria.
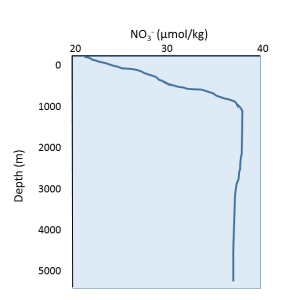
Comparisons of nutrient and dissolved oxygen profiles between the Pacific and Atlantic Oceans reveal some interesting differences (Figure 8.5.3). In general, the Atlantic has more dissolved oxygen but lower nutrient concentrations than the Pacific. Water masses form in the North Atlantic that are very cold and dense, so the water sinks to the bottom. This water will then spend the next thousand years or more moving along the seafloor from the Atlantic, to the Indian, and finally into the Pacific Ocean (see section 7.8). This water is initially oxygen-rich surface water, and as it sinks it brings oxygen to the deep seafloor. As the bottom water moves across the ocean basins, oxygen is removed through respiration and decomposition, and by the time it arrives in the Pacific it has been depleted of much of its oxygen. At the same time, decomposition of sinking organic matter adds nutrients to the deep water as it moves through the oceans, so nutrients accumulate and the Pacific water becomes nutrient-rich. Comparison of the ratios of oxygen to nutrients in the deep water can therefore provide an indication of the age of the water, i.e. how much time has passed since it initially sank from the surface in the North Atlantic. Water with a high oxygen and low nutrient content is relatively young, while older water will have less oxygen but higher nutrient concentrations.
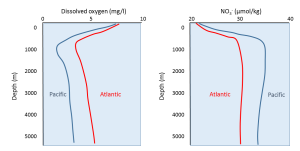
Other important nutrients, such as phosphate and silica, show similar patterns to nitrate (Figure 8.5.4), and were discussed in the section on primary production.
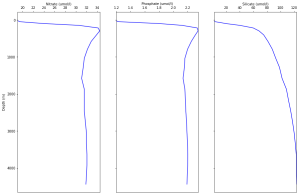
By Paul Webb, used under a CC-BY 4.0 international license. Download this book for free at https://rwu.pressbooks.pub/webboceanography/front-matter/preface/
In both hemispheres, the currents making up the western side of the gyre are much more intense than the currents on the eastern side. In other words, the currents off of the east coast of the continents are more intense than currents off of the west coast of the continents. This phenomenon is known as western intensification, and once again it is due to the Coriolis Effect.
As discussed in section 6.2, the Coriolis Effect is a result of the fact that different latitudes of the Earth are rotating at different speeds, and the apparent path taken by an object is deflected as it moves between areas of different rotation speeds. The greater the change in rotation speed, the stronger the Coriolis force. At the poles, the speed of rotation is 0 km/hr. The speed increases to about 800 km/hr at 60o latitude, 1400 km/hr at 30o latitude, and 1600 km/hr at the equator. Therefore there is an 800 km/hr difference between 60o and 90o latitude, while there is only a 200 km/hr difference between the equator and 30o. Thus the speed of Earth's rotation changes more quickly with latitude near the poles than at the equator, making the Coriolis force strongest near the poles and weakest at the equator.
The high latitude surface currents of the major gyres experience a strong Coriolis force due to their proximity to the poles. As the currents move eastward, the strong Coriolis force begins to deflect the currents towards the equator relatively early. The currents on the eastern side of the gyre are therefore spread out over a wide area as they move towards the equator (Figure 7.4.1). Near the equator, the westward flowing currents experience a much weaker Coriolis force, so their deflection does not happen until the current is all the way over to the western side of the ocean basin. These western currents must therefore move through a much narrower area (Figure 7.4.1). This imbalance means that the center of rotation of the gyre is not in the center of the ocean basins, but it closer to the western side of the gyre.
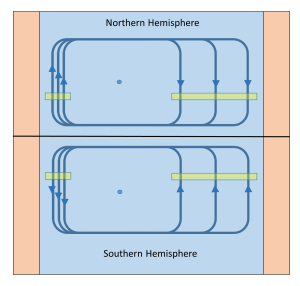
The same volume of water must pass through both the east and west sides of the gyre. In the western gyre currents, that volume is passing through a narrower area, so the current must travel faster in order to transport the same amount of water in the same amount of time. On the eastern side of the gyre the current is much wider, so the flow is slower. A simple analogy is the water flowing from a garden hose. You can make the water flow from the hose much faster and more strongly by covering part of the opening with your thumb. The same amount of water is exiting the hose whether the opening is covered or uncovered, but to get that water through the covered opening the flow has to be much faster and stronger. In the same way, western boundary currents are not only faster, but also deeper than eastern boundary currents, as they move the same volume through a narrower space. For example, the Kuroshio Current in the western Pacific is around 15 times faster, 20 times narrower, and 5 times deeper than the California Current in the eastern Pacific.
Estuaries are partially enclosed bodies of water where the salt water is diluted by fresh water input from land, creating brackish water with a salinity somewhere between fresh water and normal seawater. Estuaries include many bays, inlets, and sounds, and are often subject to large temperature and salinity variations due to their enclosed nature and smaller size compared to the open ocean.
Estuaries can be classified geologically into four basic categories based on their method of origin. In all cases they are a result of rising sea level over the last 18,000 years, beginning with the end of the last ice age; a period that has seen a rise of about 130 m. The rise in sea level has flooded coastal areas that were previously above water, and prevented the estuaries from being filled in by all of the sediments that have been emptied into them.
The first type is a coastal plain estuary, or drowned river valley. These estuaries are formed as sea level rises and floods an existing river valley, mixing salt and fresh water to create the brackish conditions where the river meets the sea. These types of estuaries are common along the east coast of the United States, including major bodies such as the Chesapeake Bay, Delaware Bay, and Narragansett Bay (Figure 4.6.1). Coastal plain estuaries are usually shallow, and since there is a lot of sediment input from the rivers, there are often a number of depositional features associated with them such as spits and barrier islands.
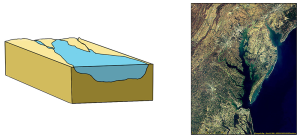
The presence of sand bars, spits, and barrier islands can lead to bar-built estuaries, where a barrier is created between the mainland and the ocean. The water that remains inside the sand bar is cut off from complete mixing with the ocean, and receives freshwater input from the mainland, creating estuarine conditions (Figure 4.6.2).
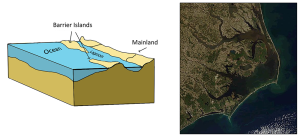
Fjords are estuaries formed in deep, U-shaped basins that were carved out by advancing glaciers. When the glaciers melted and retreated, sea level rose and filled these troughs, creating deep, steep-walled fjords (Figure 4.6.3). Fjords are common in Norway, Alaska, Canada, and New Zealand, where there are mountainous coastlines once covered by glaciers.
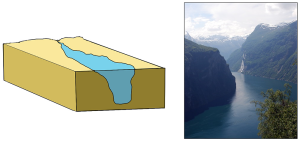
Tectonic estuaries are the result of tectonic movements, where faulting causes some sections of the crust to subside, and those lower elevation sections then get flooded with seawater. San Francisco Bay is an example of a tectonic estuary (Figure 4.6.4).
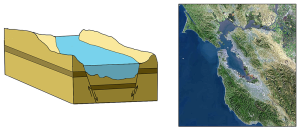
Estuaries are also classified based on their salinity and mixing patterns. The amount of mixing of fresh and salt water in an estuary depends on the rate at which fresh water enters the head of the estuary from river input, and the amount of seawater that enters the estuary mouth as a result of tidal movements. The input of fresh water is reflected in the flushing time of the estuary. This refers to the time it would take for the in-flowing fresh water to completely replace all the fresh water currently in the estuary. Seawater input is measured by the tidal volume, or tidal prism, which is the average volume of sea water entering and leaving the estuary during each tidal cycle. In other words, it is the volume difference between high and low tides. The interaction between the flushing time, tidal volume, and the shape of the estuary will determine the extent and type of water mixing within the estuary.
In a vertically mixed, or well-mixed estuary there is complete mixing of fresh and salt water from the surface to the bottom. In a particular location the salinity is constant at all depths, but across the estuary the salinity is lowest at the head where the fresh water enters, and is highest at the mouth, where the seawater comes in. This type of salinity profile usually occurs in shallower estuaries, where the shallow depths allow complete mixing from the surface to the bottom.
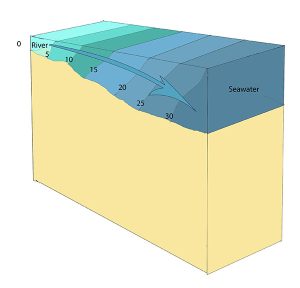
Slightly stratified or partially mixed estuaries have similar salinity profiles to vertically mixed estuaries, where salinity increases from the head to the mouth, but there is also a slight increase in salinity with depth at any point. This usually occurs in deeper estuaries than those that are well-mixed, where waves and currents mix the surface water, but the mixing may not extend all the way to the bottom.
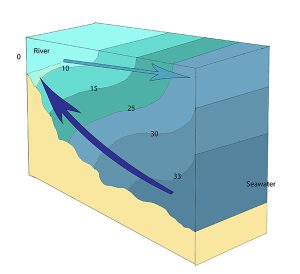
A salt wedge estuary occurs where the outflow of fresh water is strong enough to prevent the denser ocean water to enter through the surface, and where the estuary is deep enough that surface waves and turbulence have little mixing effect on the deeper water. Fresh water flows out along surface, salt water flows in at depth, creating a wedge shaped lens of seawater moving along the bottom. The surface water may remain mostly fresh throughout the estuary if there is no mixing, or it can become brackish depending on the level of mixing that occurs.
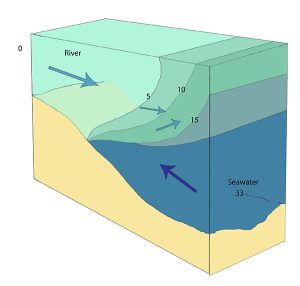
Highly stratified profiles are found in very deep estuaries, such as in fjords. Because of the depth, mixing of fresh and salt water only occurs near the surface, so in the upper layers salinity increases from the head to the mouth, but the deeper water is of standard ocean salinity.

Estuaries are very important commercially, as they are home to the majority of the world’s metropolitan areas, they serve as ports for industrial activity, and a large percentage of the world's population lives near estuaries. Estuaries are also very important biologically, especially in their role as the breeding grounds for many species of fish, birds, and invertebrates.
By Paul Webb, used under a CC-BY 4.0 international license. Download this book for free at https://rwu.pressbooks.pub/webboceanography/front-matter/preface/
Estuaries are partially enclosed bodies of water where the salt water is diluted by fresh water input from land, creating brackish water with a salinity somewhere between fresh water and normal seawater. Estuaries include many bays, inlets, and sounds, and are often subject to large temperature and salinity variations due to their enclosed nature and smaller size compared to the open ocean.
Estuaries can be classified geologically into four basic categories based on their method of origin. In all cases they are a result of rising sea level over the last 18,000 years, beginning with the end of the last ice age; a period that has seen a rise of about 130 m. The rise in sea level has flooded coastal areas that were previously above water, and prevented the estuaries from being filled in by all of the sediments that have been emptied into them.
The first type is a coastal plain estuary, or drowned river valley. These estuaries are formed as sea level rises and floods an existing river valley, mixing salt and fresh water to create the brackish conditions where the river meets the sea. These types of estuaries are common along the east coast of the United States, including major bodies such as the Chesapeake Bay, Delaware Bay, and Narragansett Bay (Figure 4.6.1). Coastal plain estuaries are usually shallow, and since there is a lot of sediment input from the rivers, there are often a number of depositional features associated with them such as spits and barrier islands.
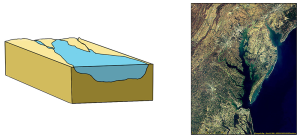
The presence of sand bars, spits, and barrier islands can lead to bar-built estuaries, where a barrier is created between the mainland and the ocean. The water that remains inside the sand bar is cut off from complete mixing with the ocean, and receives freshwater input from the mainland, creating estuarine conditions (Figure 4.6.2).
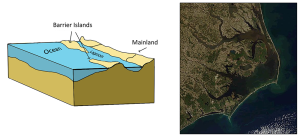
Fjords are estuaries formed in deep, U-shaped basins that were carved out by advancing glaciers. When the glaciers melted and retreated, sea level rose and filled these troughs, creating deep, steep-walled fjords (Figure 4.6.3). Fjords are common in Norway, Alaska, Canada, and New Zealand, where there are mountainous coastlines once covered by glaciers.
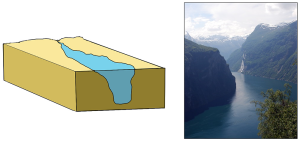
Tectonic estuaries are the result of tectonic movements, where faulting causes some sections of the crust to subside, and those lower elevation sections then get flooded with seawater. San Francisco Bay is an example of a tectonic estuary (Figure 4.6.4).
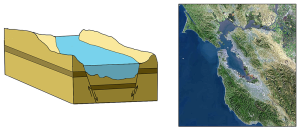
Estuaries are also classified based on their salinity and mixing patterns. The amount of mixing of fresh and salt water in an estuary depends on the rate at which fresh water enters the head of the estuary from river input, and the amount of seawater that enters the estuary mouth as a result of tidal movements. The input of fresh water is reflected in the flushing time of the estuary. This refers to the time it would take for the in-flowing fresh water to completely replace all the fresh water currently in the estuary. Seawater input is measured by the tidal volume, or tidal prism, which is the average volume of sea water entering and leaving the estuary during each tidal cycle. In other words, it is the volume difference between high and low tides. The interaction between the flushing time, tidal volume, and the shape of the estuary will determine the extent and type of water mixing within the estuary.
In a vertically mixed, or well-mixed estuary there is complete mixing of fresh and salt water from the surface to the bottom. In a particular location the salinity is constant at all depths, but across the estuary the salinity is lowest at the head where the fresh water enters, and is highest at the mouth, where the seawater comes in. This type of salinity profile usually occurs in shallower estuaries, where the shallow depths allow complete mixing from the surface to the bottom.
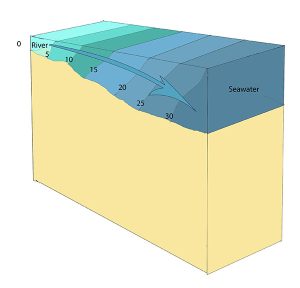
Slightly stratified or partially mixed estuaries have similar salinity profiles to vertically mixed estuaries, where salinity increases from the head to the mouth, but there is also a slight increase in salinity with depth at any point. This usually occurs in deeper estuaries than those that are well-mixed, where waves and currents mix the surface water, but the mixing may not extend all the way to the bottom.
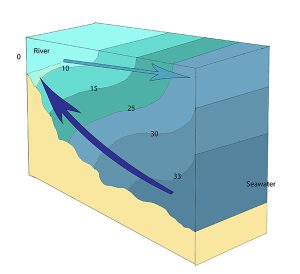
A salt wedge estuary occurs where the outflow of fresh water is strong enough to prevent the denser ocean water to enter through the surface, and where the estuary is deep enough that surface waves and turbulence have little mixing effect on the deeper water. Fresh water flows out along surface, salt water flows in at depth, creating a wedge shaped lens of seawater moving along the bottom. The surface water may remain mostly fresh throughout the estuary if there is no mixing, or it can become brackish depending on the level of mixing that occurs.

Highly stratified profiles are found in very deep estuaries, such as in fjords. Because of the depth, mixing of fresh and salt water only occurs near the surface, so in the upper layers salinity increases from the head to the mouth, but the deeper water is of standard ocean salinity.

Estuaries are very important commercially, as they are home to the majority of the world’s metropolitan areas, they serve as ports for industrial activity, and a large percentage of the world's population lives near estuaries. Estuaries are also very important biologically, especially in their role as the breeding grounds for many species of fish, birds, and invertebrates.
By Paul Webb, used under a CC-BY 4.0 international license. Download this book for free at https://rwu.pressbooks.pub/webboceanography/front-matter/preface/
The movement of surface currents also plays a role in the vertical movements of deeper water, mixing the upper water column. Upwelling is the process that brings deeper water to the surface, and its major significance is that it brings nutrient-rich deep water to the nutrient-deprived surface, stimulating primary production (see section 8.3). Downwelling is where surface water is forced downwards, where it may deliver oxygen to deeper water. Downwelling leads to reduced productivity, as it extends the depth of the nutrient-limited layer.
Upwelling occurs where surface currents are diverging, or moving away from each other. As the surface waters diverge, deeper water must be brought to the surface to replace it, creating upwelling zones. The upwelled water is cold and rich in nutrients, leading to high productivity. Many of the most productive regions on Earth are found in upwelling zones. In the equatorial Pacific, the trade winds blow the North and South Equatorial Currents towards the west, while Ekman transport causes the upper layers to move to the north and south in their respective hemispheres. This creates a divergence zone, and a region of upwelling and high productivity (Figure 7.5.1).
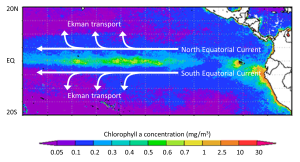
A similar process occurs near the Antarctic continent, creating one of the most productive regions on Earth, the Antarctic divergence. In this case, the West Wind Drift (Antarctic Circumpolar Current) is flowing parallel to, but in the opposite direction of the East Wind Drift. With both currents occurring in the Southern Hemisphere, Ekman transport will be to the left, so the eastward-flowing West Wind Drift water will be transported to the north, and the westward-flowing East Wind Drift water will be transported to the south, creating a highly productive divergence zone (Figure 7.5.2).

Downwelling occurs where surface currents converge. The converging water has nowhere to go but down, so the surface water sinks. Since surface water is usually low in nutrients, downwelling leads to low productivity zones. An example of a downwelling region is off of the Labrador coast in Canada, where the Gulf Stream, Labrador, and East Greenland Currents converge.
Coastal Upwelling
Upwelling and downwelling also occur along coasts, when winds move water towards or away from the coastline. Surface water moving away from land leads to upwelling, while downwelling occurs when surface water moves towards the land. Historically, some of the most productive commercial fishing grounds have been associated with coastal upwelling. Along the coast of California, the local prevailing winds blow towards the south. Ekman transport moves the surface layer 90o to the right of the wind, meaning the net Ekman transport is in an offshore direction. The water displaced near the coast is replaced by cold, nutrient-rich deeper water that is brought to the surface through upwelling, leading to high productivity (Figure 7.5.3).

The same process happens off of the coast of Peru, which for a long time had the world's largest commercial fishery. Winds along the Peruvian coast blow towards the north, and since Peru is in the Southern Hemisphere, the Ekman transport is 90o to the left of the wind, which causes the surface water to move offshore and leads to upwelling and productivity. In any coastal upwelling location, if the winds reverse, surface water moves towards the shore and downwelling is the result.
Upwelling can also occur due to geological features of the ocean floor. For example, as deep water currents encounter seamounts or other raised features, the water is forced upwards, bringing nutrient-rich water to the surface. This helps explain why productivity is often high in the water over seamounts.
Some coastal areas are dominated by erosion, an example being the Pacific coast of North America, while others are dominated by deposition, examples being the Atlantic and Caribbean coasts of the United States. But on almost all coasts, both deposition and erosion are happening to varying degrees most of the time, although in different places. On deposition-dominant coasts, the coastal sediments are still being eroded from some areas and deposited in others.
On coasts that are dominated by depositional processes, most of the sediment being deposited typically comes from large rivers. Much of the sediment is immediately deposited at the mouth of the river, creating large fan-shaped deltas. An obvious example is where the Mississippi River flows into the Gulf of Mexico at New Orleans; another is the Yellow (Huang He) River in China (Figure 4.4.1).
Figure 4.4.1 The Yellow River delta in China, created by one of the most sediment-laden rivers on Earth (NASA [Public domain], via Wikimedia Commons).
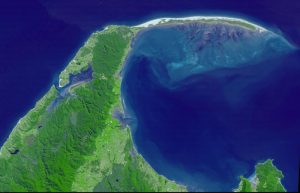
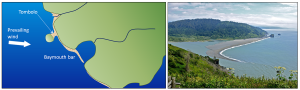
Tombolos are common where islands are abundant, and they typically form where there is a wave shadow behind a nearshore island (Figure 4.4.4). This becomes an area with reduced energy, and so the longshore current slows and sediments accumulate. Eventually enough sediments accumulate to connect the island to the mainland with a tombolo (Figure 4.4.5).
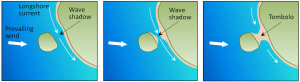
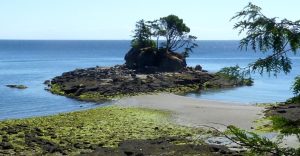
In areas where coastal sediments are abundant and coastal relief is low (because there has been little or no recent coastal uplift), it is common for barrier islands to form (Figure 4.4.6). Barrier islands are elongated islands composed of sand that form offshore from the mainland, potentially reaching several kilometers wide and hundreds of kilometers long. They are common along the U.S. Gulf Coast from Texas to Florida, and along the U.S. Atlantic Coast from Florida to Massachusetts. The islands often form as the result of sediment moving offshore through river discharge, while wave action works to push the sediment back towards the shore. The resulting sediment buildup is then stretched into long barrier islands by longshore transport.

Mature barrier islands contain a number of ecological zones. Beginning on the ocean side of the island there is a beach, consisting of the zones we discussed in section 4.1. Behind the beach lie dunes that are built up by sand transported by wind. The ocean side of the dunes are home to grasses and other plants which help stabilize the sand from erosion, and also help slow down the wind to allow sand to settle and accumulate. Beyond the dunes lies a more heavily vegetated barrier flat, covered by larger shrubs and trees that are tolerant to the high winds and salty conditions. As the land slopes down on the side of the island facing the mainland, the low-lying areas transition into a salt marsh or mud flat habitat, which is protected from wave action, but is influenced by tidal changes. The mud flats are colonized by grasses, which slow down the movement of water and lead to increased sediment deposition, building up the land in the marsh. Different species of grasses eventually dominate the different elevations of the salt marsh, depending on their tolerance for submersion in seawater. These salt marshes are very important habitats for many invertebrates, birds, and juvenile fish. Between the island and the mainland lies a lagoon, which usually contains brackish water from the mixing of fresh water runoff from the land and the seawater within a somewhat enclosed space. Barrier islands, although attractive locations for beach houses, are not permanent structures, and people should be wary of building on them. Over time, the erosion on the seaward side of the island, and the expansion of the marsh on the landward side, causes the island to slowly move towards the mainland, eventually closing off the lagoon. Maintaining dune grasses is one way to slow this movement, and as we will see in the next section, people have developed a number of other strategies to try to curtail the natural erosion of beaches.
By Paul Webb, used under a CC-BY 4.0 international license. Download this book for free at https://rwu.pressbooks.pub/webboceanography/front-matter/preface/
Modified from "Physical Geology" by Steven Earle used under a CC-BY 4.0 international license. Download this book for free at http://open.bccampus.ca