15 2.9 Seamounts and Hot Spots
The ocean floor is dotted with seamounts, some isolated and some in chains. Seamounts are underwater volcanoes, and most are much younger than the oceanic crust on which they formed. If a seamount gets large enough to break the ocean surface, it becomes a volcanic island. Some seamounts are formed from magma rising at a divergent boundary, and as the plates move apart, the seamounts move with them, which can result in a seamount chain. Other seamounts form from the rising magma at an ocean-ocean subduction zone; these include the Aleutians, extending from Alaska to Russia, and the Lesser Antilles in the eastern part of the Caribbean. Sometimes the crust on which an island or seamount sits will subside, taking the seamount with it. As this happens, the top of the seamount can become eroded flat, and these flat-topped seamounts are then called tablemounts or guyots.
However, some seamounts are formed far away from plate boundaries, in places where we would not usually expect much volcanic activity. Some seamounts and ocean islands are formed above a mantle plume or hot spot — a place where hot mantle material rises in a stationary and semi-permanent plume, and affects the overlying crust. Mantle plumes are thought to rise at approximately 10 times the rate of mantle convection. The ascending column may be on the order of kilometers to tens of kilometers across, but near the surface it spreads out to create a mushroom-style head that is several tens to over 100 kilometers across. Near the base of the lithosphere (the rigid part of the mantle), the mantle plume (and possibly some of the surrounding mantle material) partially melts to form magma that rises to feed volcanoes.
A great example of seamounts created from a hot spot includes the Hawaiian and Emperor Seamount island chains in the Pacific Ocean (Figure 2.9.1). The oldest of the Hawaiian/Emperor seamounts is dated at around 80 Ma, and it is situated on oceanic crust aged around 90 to 100 Ma. The volcanic rock making up these islands gets progressively younger toward the southeast, culminating with the island of Hawaii itself, which consists of rock that is almost all younger than 1 Ma. It appears that a stationary plume of hot upwelling mantle material is the source of the Hawaiian volcanism, and that the ocean crust of the Pacific Plate is moving toward the northwest over this hot spot. A seamount will be formed through volcanic activity over the hot spot, then the plate will move and displace the seamount before the hot spot produces the next seamount, and so on. In this way, over time, the seamounts are formed in chains. Near the Midway Islands, the chain takes a pronounced change in direction, from northwest-southeast for the Hawaiian Islands to nearly north-south for the Emperor Seamounts. This change is widely ascribed to a change in direction of the Pacific Plate moving over the stationary mantle plume, but it is also possible that the Hawaiian mantle plume has not actually been stationary throughout its history, and in fact moved at least 2,000 km south over the period between 81 and 45 Ma.
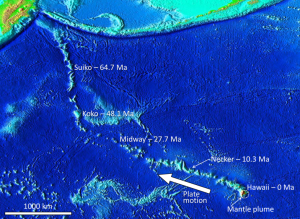
Since most mantle plumes are beneath the oceans, the early stages of volcanism typically take place on the seafloor. Over time, very large islands may form like those in Hawaii. In fact, if you measure it from its base on the seafloor to its summit, Mauna Loa on the island of Hawaii is the largest mountain on Earth, rising 9700 m (in comparison, the elevation of the summit of Mt. Everest is 8848 m). While the island of Hawaii is the youngest in the chain, there is actually a new volcano named Loihi, that is still submerged at a depth of 980 m SE of Hawaii, and may one day become a new Hawaiian island when it emerges 10,000 – 100,000 years from now.
There is evidence of many such mantle plumes around the world. Most are within the ocean basins, including places like Hawaii, Iceland, and the Galapagos Islands, but some are under continents. One example is the Yellowstone hot spot in the west-central United States, and another is the one responsible for the Anahim Volcanic Belt in central British Columbia. It is evident that mantle plumes are very long-lived phenomena, lasting for at least tens of millions of years, possibly for hundreds of millions of years in some cases.
Estuaries are partially enclosed bodies of water where the salt water is diluted by fresh water input from land, creating brackish water with a salinity somewhere between fresh water and normal seawater. Estuaries include many bays, inlets, and sounds, and are often subject to large temperature and salinity variations due to their enclosed nature and smaller size compared to the open ocean.
Estuaries can be classified geologically into four basic categories based on their method of origin. In all cases they are a result of rising sea level over the last 18,000 years, beginning with the end of the last ice age; a period that has seen a rise of about 130 m. The rise in sea level has flooded coastal areas that were previously above water, and prevented the estuaries from being filled in by all of the sediments that have been emptied into them.
The first type is a coastal plain estuary, or drowned river valley. These estuaries are formed as sea level rises and floods an existing river valley, mixing salt and fresh water to create the brackish conditions where the river meets the sea. These types of estuaries are common along the east coast of the United States, including major bodies such as the Chesapeake Bay, Delaware Bay, and Narragansett Bay (Figure 4.6.1). Coastal plain estuaries are usually shallow, and since there is a lot of sediment input from the rivers, there are often a number of depositional features associated with them such as spits and barrier islands.
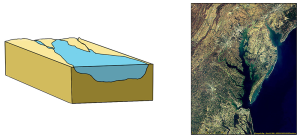
The presence of sand bars, spits, and barrier islands can lead to bar-built estuaries, where a barrier is created between the mainland and the ocean. The water that remains inside the sand bar is cut off from complete mixing with the ocean, and receives freshwater input from the mainland, creating estuarine conditions (Figure 4.6.2).

Fjords are estuaries formed in deep, U-shaped basins that were carved out by advancing glaciers. When the glaciers melted and retreated, sea level rose and filled these troughs, creating deep, steep-walled fjords (Figure 4.6.3). Fjords are common in Norway, Alaska, Canada, and New Zealand, where there are mountainous coastlines once covered by glaciers.

Tectonic estuaries are the result of tectonic movements, where faulting causes some sections of the crust to subside, and those lower elevation sections then get flooded with seawater. San Francisco Bay is an example of a tectonic estuary (Figure 4.6.4).

Estuaries are also classified based on their salinity and mixing patterns. The amount of mixing of fresh and salt water in an estuary depends on the rate at which fresh water enters the head of the estuary from river input, and the amount of seawater that enters the estuary mouth as a result of tidal movements. The input of fresh water is reflected in the flushing time of the estuary. This refers to the time it would take for the in-flowing fresh water to completely replace all the fresh water currently in the estuary. Seawater input is measured by the tidal volume, or tidal prism, which is the average volume of sea water entering and leaving the estuary during each tidal cycle. In other words, it is the volume difference between high and low tides. The interaction between the flushing time, tidal volume, and the shape of the estuary will determine the extent and type of water mixing within the estuary.
In a vertically mixed, or well-mixed estuary there is complete mixing of fresh and salt water from the surface to the bottom. In a particular location the salinity is constant at all depths, but across the estuary the salinity is lowest at the head where the fresh water enters, and is highest at the mouth, where the seawater comes in. This type of salinity profile usually occurs in shallower estuaries, where the shallow depths allow complete mixing from the surface to the bottom.

Slightly stratified or partially mixed estuaries have similar salinity profiles to vertically mixed estuaries, where salinity increases from the head to the mouth, but there is also a slight increase in salinity with depth at any point. This usually occurs in deeper estuaries than those that are well-mixed, where waves and currents mix the surface water, but the mixing may not extend all the way to the bottom.

A salt wedge estuary occurs where the outflow of fresh water is strong enough to prevent the denser ocean water to enter through the surface, and where the estuary is deep enough that surface waves and turbulence have little mixing effect on the deeper water. Fresh water flows out along surface, salt water flows in at depth, creating a wedge shaped lens of seawater moving along the bottom. The surface water may remain mostly fresh throughout the estuary if there is no mixing, or it can become brackish depending on the level of mixing that occurs.

Highly stratified profiles are found in very deep estuaries, such as in fjords. Because of the depth, mixing of fresh and salt water only occurs near the surface, so in the upper layers salinity increases from the head to the mouth, but the deeper water is of standard ocean salinity.

Estuaries are very important commercially, as they are home to the majority of the world’s metropolitan areas, they serve as ports for industrial activity, and a large percentage of the world's population lives near estuaries. Estuaries are also very important biologically, especially in their role as the breeding grounds for many species of fish, birds, and invertebrates.
By Paul Webb, used under a CC-BY 4.0 international license. Download this book for free at https://rwu.pressbooks.pub/webboceanography/front-matter/preface/
Marine Reptiles
Marine reptiles are cold blooded (ectothermic), meaning that their internal temperature is regulated by their surroundings and is not constant. They have scales that cover their bodies, they reproduce out of the water, and they evolved from amphibians. Today's marine reptiles include turtles, sea snakes, iguanas, and marine crocodiles.
Sea Turtles:
- 5 widely distributed tropical and subtropical species:
- Green, Hawksbill, Ridley, Leatherback and Loggerback
- All have large limbs and non-retractable heads
- All are excellent swimmers: the front limbs are flattened to act as oars, while the hind limbs work as rudders
- All grow to considerable size: the Atlantic Leatherback is the largest, growing to more than 1500 lbs and 11.5 ft long
- All are under threat of extinction
- The Green turtle is known for its long migrations, often of more than a thousand miles, between its feeding grounds and breeding areas
- Some use smell and vision, wave patterns, the angle of the sun and even celestial navigation to find a beach site close to where they were hatched
- if they survived there, then their offspring is more likely to survive
- https://www.seeturtles.org/sea-turtle-facts
Sea snakes:
- There are more than 50 species, which represent the most recently evolved group of marine reptiles
- They're found primarily in the warm oceans of the Indo-Pacific, but some can be found in the Atlantic (as seen in my photo)
- They grow to lengths of 4 to 10 feet
- Their bodies are flattened for swimming
- They breathe air, but have valves on their noses and can dive for crustaceans or shellfish
- They're truly marine except for one species that lays eggs on land – most give live birth at sea
- They also feed on small fish
- by catching and holding them in their jaws with their small teeth until their venom seeps into the wounds and kills the prey
- Their venom is among the most active of all known biological poisons
- the physiological effects are similar to those of cobra venom, only several times more toxic
- Fortunately for humans, they lack fangs to inject the venom and they are not terribly aggressive
- However, several people a year die from the bites of sea snakes, mostly fishermen who accidentally get bitten while removing them from their nets

Marine Iguanas:
- Found in the Galapagos Islands
- They're large, heavy and sluggish on land, but much more graceful in the sea
- They eat encrusting algae that they scrape from rocks with their spade-like teeth
Marine Crocodiles:
- These are occasional seafarers
- And are found mostly in the Indo-Pacific area from India to Australia
- They may grow to 30 ft in length
- They may be endangered because of predation by humans
Dr. Cristina Cardona
Most of the waves discussed in the previous section referred to deep water waves in the open ocean. But what happens when these waves move towards shore and encounter shallow water? Remember that in deep water, a wave’s speed depends on its wavelength, but in shallow water wave speed depends on the depth (section 3.1). When waves approach the shore they will "touch bottom" at a depth equal to half of their wavelength; in other words, when the water depth equals the depth of the wave base (Figure 3.3.1). At this point their behavior will begin to be influenced by the bottom.
When the wave touches the bottom, friction causes the wave to slow down. As one wave slows down, the one behind it catches up to it, thus decreasing the wavelength. However, the wave still contains the same amount of energy, so while the wavelength decreases, the wave height increases. Eventually the wave height exceeds 1/7 of the wavelength, and the wave becomes unstable and forms a breaker. Often breakers will start to curl forwards as they break. This is because the bottom of the wave begins to slow down before the top of the wave, as it is the first part to encounter the seafloor. So the crest of the wave gets “ahead” of the rest of the wave, but has no water underneath it to support it (Figure 3.3.1).
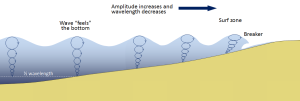
There are three main types of breakers: spilling, plunging, and surging. These are related to the steepness of the bottom, and how quickly the wave will slow down and its energy will get dissipated.
- Spilling breakers form on gently sloping or flatter beaches, where the energy of the wave is dissipated gradually. The wave slowly increases in height, then slowly collapses on itself (Figure 3.3.2). For surfers, these waves provide a longer ride, but they are less exciting.
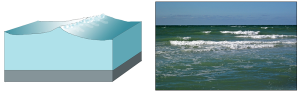
- Plunging breakers form on more steeply-sloped shores, where there is a sudden slowing of the wave and the wave gets higher very quickly. The crest outruns the rest of the wave, curls forwards and breaks with a sudden loss of energy (Figure 3.3.3). These are the “pipeline” waves that surfers seek out.
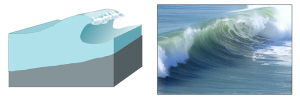
- Surging breakers form on the steepest shorelines. The wave energy is compressed very suddenly right at the shoreline, and the wave breaks right onto the beach (Figure 3.3.4). These waves give too short (and potentially painful) a ride for surfers to enjoy.
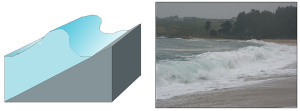
Wave Refraction
Swell can be generated anywhere in the ocean and therefore can arrive at a beach from almost any direction. But if you have ever stood at the shore you have probably noticed that the waves usually approach the shore somewhat parallel to the coast. This is due to wave refraction. If a wave front approaches shore at an angle, the end of the wave front closest to shore will touch bottom before the rest of the wave. This will cause that shallower part of the wave to slow down first, while the rest of the wave that is still in deeper water will continue on at its regular speed. As more and more of the wave front encounters shallower water and slows down, the wave font refracts and the waves tend to align themselves nearly parallel to the shoreline (they are refracted towards the region of slower speed). As we will see in section 5.2, the fact that the waves do not arrive perfectly parallel to the beach causes longshore currents and longshore transport that run parallel to the shore.
Refraction can also explain why waves tend to be larger off of points and headlands, and smaller in bays. A wave front approaching shore will touch the bottom off of the point before it touches bottom in a bay. Once again, the shallower part of the wave front will slow down, and cause the rest of the wave front to refract towards the slower region (the point). Now all of the initial wave energy is concentrated in a relatively small area off of the point, creating large, high energy waves (Figure 3.3.6). In the bay, the refraction has caused the wave fronts to refract away from each other, dispersing the wave energy, and leading to calmer water and smaller waves. This makes the large waves of a “point break” ideal for surfing, while water is calmer in a bay, which is where people would launch a boat. This difference in wave energy also explains why there is net erosion on points, while sand and sediments get deposited in bays (see section 5.3).
By Paul Webb, used under a CC-BY 4.0 international license. Download this book for free at https://rwu.pressbooks.pub/webboceanography/front-matter/preface/