9 2.3 Mechanisms for Plate Motion
In section 2.1 we learned that one of the reasons that Wegener’s ideas of continental drift were initially rejected by the scientific community was that he could not provide a plausible mechanism for plate motion. However, with all that we have learned about the processes occurring in the Earth’s interior since then, there is still some debate about the actual forces that make the plates move. One side in the argument holds that the plates are only moved by the traction caused by mantle convection. The other side holds that traction plays only a minor role and that two other forces, ridge push and slab pull, are more important. Some argue that the real answer lies somewhere in between.
To understand mantle convection, imagine a pot of water on a hot stove. The water at the bottom of the pot near the heat source becomes hot and expands, making it lighter (less dense) than the water above. The hot, low density water rises, and cooler, denser water sinks and flows in from the sides. This water then gets heated and rises, and the cycle continues. This creates a circular pattern of rising and sinking water called a convection cell. (To test this, try sprinkling a few flakes of spice in the center of a rapidly boiling pot of water. The flakes will move outwards to the edge of the pot as warmer water rises and pushes them aside).
Heat is continuously flowing outward from Earth’s interior, and the transfer of heat from the core to the mantle causes convection in the mantle (Figure 2.3.1). Even though the mantle material is essentially solid rock, it is sufficiently plastic (fluid) to slowly flow (at rates of centimeters per year) as long as a steady force is applied to it. This convection is a driving force for the movement of tectonic plates, as the horizontal movements of mantle under the crust drag the plates with them. At places where convection currents in the mantle are moving upward, new lithosphere forms and the plates move apart (diverge). Where two plates are converging (and the convective flow is downward), one plate will be subducted (pushed down) into the mantle beneath the other.
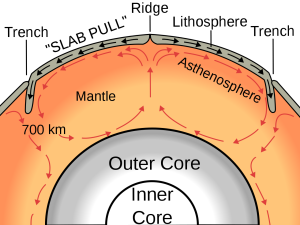
The ridge push/slab pull model also relies on mantle convection, but in this case it is not simply the traction from the convection cell that moves the plates. In this model, plates move through a combination of pull from the weight of the subducting edge of the plates, and through the outward pushing of an ocean ridge where magma is rising and forming new crust (Figure 2.3.2).
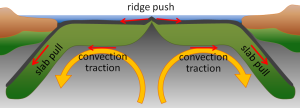
Additional links for more information:
- Animation speculating on the movements of the continents over the last 3 billion years…. https://www.youtube.com/watch?v=UwWWuttntio
- And what might happen over the next 300 million years: https://www.youtube.com/watch?v=bQywDr-btz4

Marine Reptiles
Marine reptiles are cold blooded (ectothermic), meaning that their internal temperature is regulated by their surroundings and is not constant. They have scales that cover their bodies, they reproduce out of the water, and they evolved from amphibians. Today's marine reptiles include turtles, sea snakes, iguanas, and marine crocodiles.
The first paragraph was written by Keddy (University of California, Davis), is shared under a not declared license and was authored, remixed, and/or curated by LibreTexts. Download this book for free at https://geo.libretexts.org/Courses/Diablo_Valley_College/OCEAN-101%3A_Fundamentals_of_Oceanography_(Keddy)
The rest was written by Dr. Cristina Cardona.
For most people, when they think of coastal areas they picture a beach, and the beach that they imagine is probably a typical sandy beach composed of quartz sand grains. But beaches are comprised of whatever types of sediments are dominant in the local area. For example, parts of Hawaii and Iceland are famous for their black sand beaches, made up of eroded basalt and other volcanic materials. The beautiful tropical white sand beaches we see in travel ads are largely composed of the crushed calcium carbonate remains of coral skeletons (much of which has been chewed up and excreted by a fish before we happily run our toes through it!) Other beaches may lack sand altogether and instead be dominated by small shells, or larger rocks or pebbles (Figure 4.1.1).
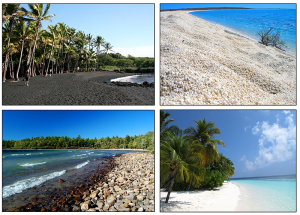
The shoreline is divided up into multiple zones (Figure 4.1.2). The backshore is the region of the beach above the high tide line, which is only submerged under unusually high wave conditions, such as during storms. The foreshore lies between the high tide and low tide lines; it is submerged during high tide and is exposed during low tide. The nearshore extends from the low tide line to the depth where wave action is no longer influenced by the bottom, i.e. to where the depth exceeds the wave base (section 3.1). Finally, the offshore zone represents the depths beyond the nearshore region.
Along the beach itself, the area above the high tide line is called the berm, which is usually dry and relatively flat. The berm often ends with a berm crest or berm scarp, which is a steeper wall carved out by wave action that leads down to the foreshore. The foreshore has a number of other names, including the beach face, the intertidal or littoral zone, and if the area is fairly flat, the low tide terrace. Just off shore from the beach there are often longshore bars and longshore troughs running parallel to the beach. The longshore bars are accumulations of sand that are deposited by wave action and longshore currents (section 4.2). The decrease in depth above longshore bars is what often causes waves to start to break well before reaching the beach (section 3.3).

The sand or other particles that make up the beach are distributed by wave action. The water that moves over a beach through incoming waves is called swash, and it also contains suspended sand grains that can get deposited on the beach. Some of the swash percolates into the sand while the rest of the water washes back out as backwash as the wave recedes. Backwash removes sand from the beach and returns it to the ocean. Sand will therefore be deposited or eroded depending on which process is dominant. If wave action is light, a lot of incoming water gets absorbed by the sand, so swash dominates. Under heavier waves the beach becomes saturated with water, so less can be absorbed, and backwash is dominant. This leads to seasonal cycles in beach structure; waves are heavier during the winter as a result of stormier conditions at sea, so backwash dominates and sand is removed from the beach and deposited offshore in longshore bars. In the summer the waves are gentler, swash dominates, and the sand is transported from the longshore bar and deposited on the shore to create a wider, sandy beach (Figure 4.1.3).

By Paul Webb, used under a CC-BY 4.0 international license. Download this book for free at https://rwu.pressbooks.pub/webboceanography/front-matter/preface/

Suborder Pinnipedia: seals, sea lions and walruses
- As a group, they are fairly young, having been around for only about 25 million years
- They consist of three families:
- Otariidae: sea lions
- Phocidae: true seals
- Odobenidae: walruses
Otariidae (sea lions) have:
- small, but obvious external ears
- long, flexible necks
- independent and mobile hind limbs that permit substantial movement on land
- nostrils that are located at the tip of the snout
- a dense and soft undercoat of fur protected by a layer of long and close guard hairs
- several layers of blubber for thermal protection
Phocidae (true seals) differ from sea lions in that they have:
- no external ears
- smooth, coarse hair with no underfur
- a hind limb geometry that restricts their ability to move on land
- fore flippers that are smaller than those of sea lions
Diving behavior and adaptations:
- can shut off their nostrils completely while diving
- can restrict the flow of blood to vital organs
- can store lots of oxygen
- routinely dive for 30 minutes
Odobenidae (Walruses):
- These creatures are limited to the Arctic waters of the Atlantic and Pacific
- Their hind limbs are similar to those of sea lions, but they have no external ear
- The most characteristic feature is the set of enlarged canine teeth – tusks
- They may weigh up to 4000 pounds
- They have air sacs in their cheeks that are used for support like a life preserver because they sleep in the water
- They also use their tusks to hoist themselves out of the water onto the ice
- They are becoming rare; estimates of their numbers world-wide are around 170,000 to 190,000 individuals
Suborder Fissipedia: sea otters
- These members of the weasel family are included in the list of marine mammals because they do live in the sea and are obviously mammals
- They have the distinction of having the most valuable fur of any animal – their dark brown underfur is extraordinarily dense and rich
- Trade in sea otter fur dates back at least to 1786, under the Spanish
- This became the most important industry of the California coast and most otters were gone by 1850
- In 1900, a small population was discovered near Bixby Creek on the central California coast
- Today, the otter's range has expanded along much of the Pacific coast of the U.S., especially since their protection under the Marine Mammal Protection Act of 1972
- They grow to lengths of about 5 ft and weights up to about 80 pounds
- They live for up to about 20 years
- They live by foraging for urchins, abalone, mussels, clams, crabs and other tidbits in relatively shallow water, rarely diving deeper than 60 ft
- They frequently consume their food in an attitude of leisure as they float gently on their backs
- They will use stones to smash open shellfish by placing them on their stomachs as they float on their backs
- They have very high metabolic rates and must consume up to 25% of their body weight each day in order to maintain an internal temperature of 100 degrees
- This works out to be about 140 to 190 calories per kilogram of body weight per day, compared to the average human who must consume about 32 calories per kilogram of body weight per day
- If a sea otter doesn't eat for a day, it will lose up to 10% of its body weight
- They live in the kelp forests and use the kelp as a tether while resting at the surface
- They help protect the kelp forest by eating the sea urchins that feed on the kelp
- They also use the kelp as protection from their enemies, including sharks and killer whales
- Making them a keystone species in this habitat
Order Sirenia: sea cows
- This group includes the manatees, which are found in the shallow bays and estuaries of North America and Africa, and the dugongs, which are found in the Indo-Pacific regions
- They're common in the winter in the warm-springs of Florida's west coast including the Crystal River
- These lovable rascals feed on water plants (especially the hyacinths in southern canals) – they're strictly vegetarians
- They show little fear of man and, as a result, many are killed or severely injured each year by boat propellers, as they have a habit of swimming just below the surface
- They form family groups and appear to show great care and affection for family members
The Sirenians were named after the Sirens, the mermaids that Ulysses encountered on his return from the Trojan wars – apparently they resembled mermaids to sailors who had been too long at sea or were drunk (or maybe both)...
In the images below, can you tell which is the real mermaid?
Written by Dr. Cristina Cardona.
Most of the waves discussed in the previous section referred to deep water waves in the open ocean. But what happens when these waves move towards shore and encounter shallow water? Remember that in deep water, a wave’s speed depends on its wavelength, but in shallow water wave speed depends on the depth (section 3.1). When waves approach the shore they will "touch bottom" at a depth equal to half of their wavelength; in other words, when the water depth equals the depth of the wave base (Figure 3.3.1). At this point their behavior will begin to be influenced by the bottom.
When the wave touches the bottom, friction causes the wave to slow down. As one wave slows down, the one behind it catches up to it, thus decreasing the wavelength. However, the wave still contains the same amount of energy, so while the wavelength decreases, the wave height increases. Eventually the wave height exceeds 1/7 of the wavelength, and the wave becomes unstable and forms a breaker. Often breakers will start to curl forwards as they break. This is because the bottom of the wave begins to slow down before the top of the wave, as it is the first part to encounter the seafloor. So the crest of the wave gets “ahead” of the rest of the wave, but has no water underneath it to support it (Figure 3.3.1).
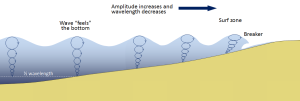
There are three main types of breakers: spilling, plunging, and surging. These are related to the steepness of the bottom, and how quickly the wave will slow down and its energy will get dissipated.
- Spilling breakers form on gently sloping or flatter beaches, where the energy of the wave is dissipated gradually. The wave slowly increases in height, then slowly collapses on itself (Figure 3.3.2). For surfers, these waves provide a longer ride, but they are less exciting.

- Plunging breakers form on more steeply-sloped shores, where there is a sudden slowing of the wave and the wave gets higher very quickly. The crest outruns the rest of the wave, curls forwards and breaks with a sudden loss of energy (Figure 3.3.3). These are the “pipeline” waves that surfers seek out.

- Surging breakers form on the steepest shorelines. The wave energy is compressed very suddenly right at the shoreline, and the wave breaks right onto the beach (Figure 3.3.4). These waves give too short (and potentially painful) a ride for surfers to enjoy.

Wave Refraction
Swell can be generated anywhere in the ocean and therefore can arrive at a beach from almost any direction. But if you have ever stood at the shore you have probably noticed that the waves usually approach the shore somewhat parallel to the coast. This is due to wave refraction. If a wave front approaches shore at an angle, the end of the wave front closest to shore will touch bottom before the rest of the wave. This will cause that shallower part of the wave to slow down first, while the rest of the wave that is still in deeper water will continue on at its regular speed. As more and more of the wave front encounters shallower water and slows down, the wave font refracts and the waves tend to align themselves nearly parallel to the shoreline (they are refracted towards the region of slower speed). As we will see in section 5.2, the fact that the waves do not arrive perfectly parallel to the beach causes longshore currents and longshore transport that run parallel to the shore.
Refraction can also explain why waves tend to be larger off of points and headlands, and smaller in bays. A wave front approaching shore will touch the bottom off of the point before it touches bottom in a bay. Once again, the shallower part of the wave front will slow down, and cause the rest of the wave front to refract towards the slower region (the point). Now all of the initial wave energy is concentrated in a relatively small area off of the point, creating large, high energy waves (Figure 3.3.6). In the bay, the refraction has caused the wave fronts to refract away from each other, dispersing the wave energy, and leading to calmer water and smaller waves. This makes the large waves of a “point break” ideal for surfing, while water is calmer in a bay, which is where people would launch a boat. This difference in wave energy also explains why there is net erosion on points, while sand and sediments get deposited in bays (see section 5.3).

By Paul Webb, used under a CC-BY 4.0 international license. Download this book for free at https://rwu.pressbooks.pub/webboceanography/front-matter/preface/
Written by Dr. Cristina Cardona.
Large marine disasters involving petroleum spills have happened many times over the past century. The impact of these events depends on where and how they occur. Some are accidents, others include intentional acts of war, such as the destruction of Kuwait’s oil fields in the First Gulf War. Oil spills can have a wide variety of impacts ranging from minimal (when far from coastal regions) to catastrophic when they impact shore regions. Two of the largest (most expensive) petroleum-related disasters affecting North American coastal waters are discussed below.
The Deepwater Horizon Disaster, 2010
The Deepwater Horizon Disaster started on April 20, 2010 with an explosion and fire of a submersible drilling platform located in the Gulf of Mexico about 40 miles (64 km) offshore from the Louisiana coast. The drilling operation involved tapping an oil reservoir deep in sedimentary deposits on the offshore region beyond the continental shelf. Problems with the drilling operation and failure of equipment to prevent a blowout that resulted in the explosion and fire, and the eventual sinking of the drilling platform two days later. 17 platform workers were killed and another 11 were injured by the explosion and fire. The open well, sheared of at the seabed, proceeded to spew large quantities of crude oil into Gulf waters until it was shut down in mid July when a 75 ton cap was put in place, sealing off the well. The disaster resulted in the largest oil spill in the history of the Petroleum Industry.
An estimated 200 million gallons (about 5 million barrels) of oil poured into ocean from the unconstrained well. Some of oil stayed on or near the seabed, much of it formed a large plum in layers within the ocean waters, and some migrated to the surface where wind and currents dispersed it. An extensive cleanup effort was undertaken to trap, degrade and disperse, or burn off much of the oil on the surface. Unfortunately, large amounts found its way onshore, impacting beaches and coastal wetlands, and severely impacting wildlife. The bad publicity wreaked economic havoc on coastal communities and businesses involved in fishing and recreation from Texas to Florida. As of 2015, BP (the company that operated the drilling program) agreed to pay $18.7 billion to settle all federal and state claims for the disaster - the biggest pollution penalty in U.S. history. Total settlement costs was in the range of $54 billion.
Settlement of all federal and state claims brings total costs to nearly $54 billion. BP PLC agreed to pay $18.7 billion to settle all federal and state claims arising from the 2010 Deepwater Horizon oil spill, including the biggest pollution penalty in U.S. history.
Because the spill happened in the warm open ocean waters, much of the crude oil from the spill eventually dispersed (evaporated or diluted) or was consumed by microbial activity.
![]() |
||
Figure 12.10.2. An oil slick spreads on the Gulf of Mexico from the Deep Horizon disaster. |
Exxon Valdez Oil Spill Disaster, 1989
Prior to the Deepwater Horizon disaster, the worst petroleum-related disaster affecting the US coastline was the Exxon Valdez oil spill (Figures 17-24 and 17-25). The oil spill occurred in Prince William Sound in the Gulf of Alaska. The Exxon Valdez, a large oil tanker bound for refineries in Long Beach, California, veered off course and struck a submerged rock “reef” outcrop on March 24, 1989. The disaster was blamed on poor navigation by a drunken ship captain, poorly trained personnel, and faulty and unused navigation equipment.
Estimates by governmental and other sources suggest that at least 10 to 11 million gallons (about 250,000 barrels) of Alaskan crude oil spilled into the coastal waters. The spill, so close to shore, eventually impaction about 1,300 miles (2,000 km) of coastline in the Gulf of Alaska (closer to 9,000 miles [14,500 km] considering all the islands, headlands, and bays along the rugged coastline). Rough seas, and the rugged and remote coastline made clean-up efforts extremely difficult, and the cold-water setting hampered the rapid decay and dispersion of the oil. The spill devastated habitats for salmon, seals, seabirds, and sea otters, and had a catastrophic effect of coastal communities in the region. The cost of the disaster, spread over many years, was in the range of about $7 billion. Hard facts were learned from the disaster. It turns out that some of the beach areas were "cleaned" - basically cooked with 150 F water. These areas were actually harmed more by the cleaning processes used. It was determined that about 35% of the oil evaporated, 8% burned, 5% dispersed by surf , and only about 5% biodegraded; the rest formed slicks that dispersed into the greater ocean currents offshore.
An outcome of the disaster is that all new large petroleum-transport vessels are now being built with double hulls to hopefully prevent future transport-spill disasters.
Impact of Petroleum Pollution on Wildlife
Pollution from petroleum-source products is a major problem in parts of the worlds oceans and coastlines. In addition, tar-ball, waxes, and other petroleum-derivative products can now be found throughout the world’s oceans. Evidence of the pollution is most abundant along developed (urban and industrial) coastlines where accidental spills occur most frequently. The risks of spills occur along all infrastructure systems associated with the petroleum production, refining, transportation, and consumption. Leaky oil from cars and trucks are a major non-point source of water pollution. Large oil spills and oil production disasters are some of the most costly, devastating both wildlife and the economic livelihoods of communities in regions where they occur (Figure 17.26).
Oil spills are particularly bad for homeothermic (warm blooded) organisms with fur of feathers. Saturation with oil causes these animals to loose insulation and they die from hypothermia. Oil slicks poison kill 150-450,000 sea birds killed each year. Organisms living in the intertidal zone most sensitive to oil contamination.
Refined oil and oil-derivative products tend to be non-biodegradable, and are more toxic to wildlife.
From Miracosta College, is shared under a not declared license and was authored, remixed, and/or curated by LibreTexts. Download this book for free at https://geo.libretexts.org/Bookshelves/Oceanography/Oceanography_101_(Miracosta)