3 1.3 Marine Provinces
In section 1.2 we learned about the regions that make up the continental margins. So before we leave this topic, we will look at some of the other ways we can categorize the ocean environments.
The first major distinction is between the pelagic and benthic zones. The pelagic zone refers to the water column, where swimming and floating organisms live. The benthic zone refers to the bottom, and organisms living on and in the bottom are known as the benthos.
The pelagic zone is divided into two provinces: the neritic province corresponds to all of the water from the low tide line to the shelf break, while the oceanic province represents all of the other water in the open ocean regions.
The oceanic province is divided into depth zones (Figure 1.3.1):
- 0-200 m is the epipelagic zone (“epi” = “upon”, as in on top of the pelagic zone). This is the region where enough light penetrates the water to support photosynthesis (see section 7.3), so it is also called the euphotic or photic zone.
- 200-1000 m is the mesopelagic zone (“meso” = “middle”). There is some light here, but not enough for photosynthesis, so it is called the dysphotic zone, or the twilight zone.
- 1000-4000 m is the bathypelagic zone (“bathy” = “deep”). There is no light at these depths, so it is referred to as the aphotic zone. About 75% of the living space in the ocean lies at these depths or deeper.
- 4000-6000 m is the abyssopelagic or abyssalpelagic zone, which extends to the seafloor in most areas.
- 6000 m and below is the hadopelagic or hadalpelagic zone (named for Hades or “hell”). This refers to the water in deep ocean trenches.
Inhabitants of these regions are referred to according to their habitat, for example mesopelagic fish, or epipelagic squid.
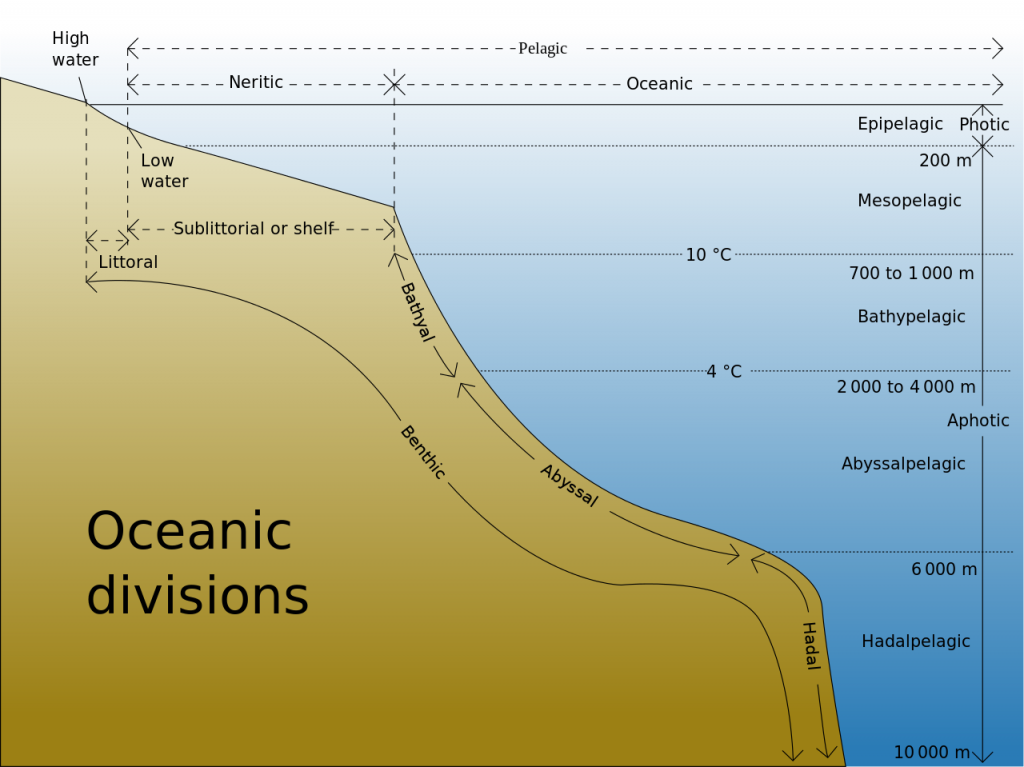
The benthic environment is also divided into zones, most of which correspond to the pelagic divisions:
- The supralittoral zone lies above the high tide line. Also called the spray zone, it is only submerged during storms or unusually high waves.
- The littoral zone is the region between the high and low tides. Thus it is also referred to as the intertidal zone.
- Below the littoral zone is the sublittoral (shelf) zone, extending from the low tide mark to the shelf break, essentially covering the continental shelf.
- The bathyal zone extends along the bottom from the shelf break to 4000m, so it generally includes the continental slope and rise.
- The abyssal zone is found between 4000-6000 m, including most of the abyssal plains. The abyssal zone represents about 80% of the benthic environment.
- The hadal zone includes all benthic regions deeper than 6000 m, such as in the bottom of trenches.
By Paul Webb, used under a CC-BY 4.0 international license. Download this book for free at https://rwu.pressbooks.pub/webboceanography/front-matter/preface/
Marine Reptiles
Marine reptiles are cold blooded (ectothermic), meaning that their internal temperature is regulated by their surroundings and is not constant. They have scales that cover their bodies, they reproduce out of the water, and they evolved from amphibians. Today's marine reptiles include turtles, sea snakes, iguanas, and marine crocodiles.
Sea Turtles:
- 5 widely distributed tropical and subtropical species:
- Green, Hawksbill, Ridley, Leatherback and Loggerback
- All have large limbs and non-retractable heads
- All are excellent swimmers: the front limbs are flattened to act as oars, while the hind limbs work as rudders
- All grow to considerable size: the Atlantic Leatherback is the largest, growing to more than 1500 lbs and 11.5 ft long
- All are under threat of extinction
- The Green turtle is known for its long migrations, often of more than a thousand miles, between its feeding grounds and breeding areas
- Some use smell and vision, wave patterns, the angle of the sun and even celestial navigation to find a beach site close to where they were hatched
- if they survived there, then their offspring is more likely to survive
- https://www.seeturtles.org/sea-turtle-facts
Sea snakes:
- There are more than 50 species, which represent the most recently evolved group of marine reptiles
- They're found primarily in the warm oceans of the Indo-Pacific, but some can be found in the Atlantic (as seen in my photo)
- They grow to lengths of 4 to 10 feet
- Their bodies are flattened for swimming
- They breathe air, but have valves on their noses and can dive for crustaceans or shellfish
- They're truly marine except for one species that lays eggs on land – most give live birth at sea
- They also feed on small fish
- by catching and holding them in their jaws with their small teeth until their venom seeps into the wounds and kills the prey
- Their venom is among the most active of all known biological poisons
- the physiological effects are similar to those of cobra venom, only several times more toxic
- Fortunately for humans, they lack fangs to inject the venom and they are not terribly aggressive
- However, several people a year die from the bites of sea snakes, mostly fishermen who accidentally get bitten while removing them from their nets

Marine Iguanas:
- Found in the Galapagos Islands
- They're large, heavy and sluggish on land, but much more graceful in the sea
- They eat encrusting algae that they scrape from rocks with their spade-like teeth

Marine Crocodiles:
- These are occasional seafarers
- And are found mostly in the Indo-Pacific area from India to Australia
- They may grow to 30 ft in length
- They may be endangered because of predation by humans
Written by Dr. Cristina Cardona.
{{unknown}}
{{unknown}}
With so many variables playing a role in the production of tides, it is understandable that not every place on Earth will experience exactly the same tidal conditions. There are three primary classifications for tides, depending on the number and relative heights of tidal cycles per day.
A diurnal tide consists of only one high tide and one low tide per day (Figure 3.7.1). "Diurnal" refers to a daily occurrence, so a situation where there is only one complete tidal cycle per day is considered a diurnal tide. Diurnal tides are common in the Gulf of Mexico, along the west coast of Alaska, and in parts of Southeast Asia.
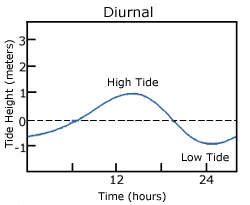
A semidiurnal tide exhibits two high and two low tides each day, with both highs and both lows of toughly equal height (Figure 3.7.2). "Semidiurnal" means "half of a day"; one tidal cycle takes half of a day, therefore there are two complete cycles per day. Semidiurnal tides are common along the east coasts of North America and Australia, the west coast of Africa, and most of Europe.
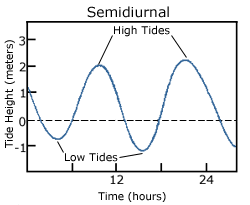
Mixed semidiurnal tides (or mixed tides), have two high tides and two low tides per day, but the heights of each tide differs; the two high tides are of different heights, as are the two low tides (Figure 3.7.3). The differences in height may be the result of amphidromic circulation, the angle of the moon, or any of the other variables discussed in section 3.6. Mixed semidiurnal tides are found along the Pacific coast of North America.
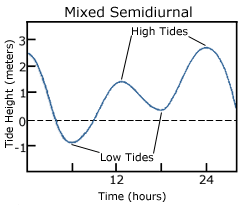
Figure 3.7.4 shows the distribution of the various tide types throughout the world.

Tidal Currents
The movement of water with the rising and falling tide creates tidal currents. As the tide rises, water flows into an area, creating a flood current. As the tide falls and water flows out an ebb current is created. Slack water, or slack tides occur during the transition between incoming high and outgoing low tides, when there is no net water movement.
The strength of a tidal current depends on the volume of water that enters and exits with each tidal cycle (the tidal volume or tidal prism), and the area through which the water flows. A large tidal volume moving through a large area may create only a weak tidal current, as the volume is spread over a wide area. On the other hand, a narrow area may produce a strong tidal current even if the tidal volume is small, as all of the water is forced through a small area. It follows that the strongest tidal currents will result from a large tidal range moving through a narrow area.
Tidal bores occur where rivers meet the ocean. If the incoming tidal current is stronger than the river outflow, the tidal bore appears as a wave, or moving wall of water that moves up the river as the tide comes in (Figure 3.7.5).

In many cases these tidal bores may move through a river or inlet for many kilometers, and if they are large enough they can form continually breaking waves that surfers can ride much farther and longer than a traditional ocean wave, such as the Severn Bore in England, shown in the video below.
https://youtu.be/IKA39LQOIck
Additional links for more information
- For an even more dramatic tidal bore, watch this video of the "Silver Dragon" on China's Qiantang River
By Paul Webb, used under a CC-BY 4.0 international license. Download this book for free at https://rwu.pressbooks.pub/webboceanography/front-matter/preface/
If you look at a map of Earth, you may notice that some of the continents seem to fit together. An early reference to this phenomenon came from Francis Bacon in the 17th century, who noticed the similarities in the Atlantic coasts of Africa, and North and South America. This apparent fit is due to the fact the continents were once connected, and have since moved apart in what has been called continental drift. However, we now know that it is not just the continents that move, so a more correct term is plate tectonics. We can credit Alfred Wegener (Figure 2.1.1) as the originator of this idea.
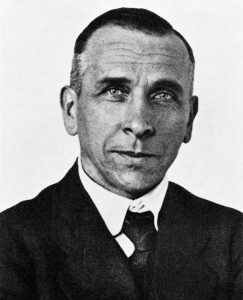
Alfred Wegener (1880-1930) earned a PhD in astronomy at the University of Berlin in 1904, but he had always been interested in geophysics and meteorology and spent most of his academic career working in meteorology. In 1911 he happened on a scientific publication that included a description of the existence of matching Permian-aged terrestrial fossils in various parts of South America, Africa, India, Antarctica, and Australia (Figure 2.1.2). Wegener concluded that this distribution of fossils could only exist if these continents were joined together. Furthermore, some of these transcontinental areas have similar fossils until around 150 million years ago, then they begin to diverge, suggesting that the areas eventually separated and speciation took different paths on the separate continents. Wegener coined the term Pangaea (“all land”) for the supercontinent from which all of the present-day continents diverged.
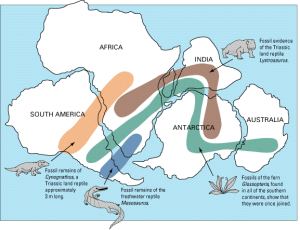
Wegener pursued his theory with determination — combing the libraries, consulting with colleagues, and making observations — looking for evidence to support it. In addition to the fit of the continents and the fossil evidence, Wegener relied heavily on matching geological patterns across oceans, such as sedimentary strata in South America matching those in Africa (Fig. 2.1.3), North American coalfields matching those in Europe, and the mountains of Atlantic Canada matching those of northern Britain both in morphology and rock type.
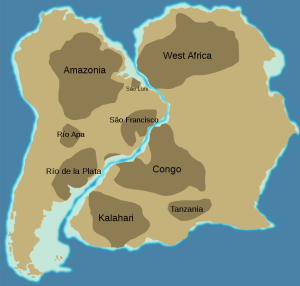
Wegener also referred to the evidence for the Carboniferous and Permian (~300 Ma) Karoo Glaciation in South America, Africa, India, Antarctica, and Australia (Fig. 2.1.4). These areas contain evidence of past glacial deposits, including glacial scars oriented away from the poles, despite the fact that some of these locations are now tropical environments. This indicates that these continents were once closer to the south pole where the glaciers could have formed. Wegener argued that this could only have happened if these continents were once all connected as a single supercontinent. He also cited evidence (based on his own astronomical observations) that showed that the continents were moving with respect to each other, and determined a separation rate between Greenland and Scandinavia of 11 m per year, although he admitted that the measurements were not accurate. In fact they weren’t even close — the separation rate is actually about 2.5 cm per year!

Wegener first published his ideas in 1912 in a short book called Die Entstehung der Kontinente (The Origin of Continents), and then in 1915 in Die Entstehung der Kontinente und Ozeane (The Origin of Continents and Oceans). He revised this book several times up to 1929, and it was translated into French, English, Spanish, and Russian. However, despite his range of evidence, the continental fits were not perfect and the geological match-ups were not always consistent (while the continental fit left some gaps when using the current coastline, it was demonstrated in the 1960s that using a 500 m depth contour gives a much tighter fit). But the most serious problem of all was that Wegener could not conceive of a good mechanism for moving the continents around. Wegener proposed that the continents were like icebergs floating on heavier crust, but the only forces that he could invoke to propel continents around were poleflucht, the effect of Earth’s rotation pushing objects toward the equator, and the lunar and solar tidal forces, which tend to push objects toward the west. It was quickly shown that these forces were far too weak to move continents, and without any reasonable mechanism to make it work, Wegener’s theory was quickly dismissed by most geologists of the day. Alfred Wegener died in Greenland in 1930 while carrying out studies related to glaciation and climate. At the time of his death, his ideas were tentatively accepted by only a small minority of geologists, and soundly rejected by most. However, within a few decades that was all to change.
Additional links for more information:
- For more about Wegener and the other pioneers of plate tectonics, visit The Geological Society's Plate Tectonics site: https://www.geolsoc.org.uk/Plate-Tectonics/Chap1-Pioneers-of-Plate-Tectonics
In the previous section we learned that rising air creates low pressure systems, and sinking air creates high pressure. In addition to their role in creating the surface winds, these high and low pressure systems also influence other climatic phenomena. Along the equator air is rising as it is warmed by solar radiation (section 6.2). Warm air contains more water vapor than cold air, which is why we experience humidity during the summer and not during the winter. The water content of air roughly doubles with every 10o C increase in temperature. So the air rising at the equator is warm and full of water vapor; as it rises into the upper atmosphere it cools, and the cool air can no longer hold as much water vapor, so the water condenses and forms rain. Therefore, low pressure systems are associated with precipitation, and we see wet habitats like tropical rainforests near the equator (Figure 6.3.1).
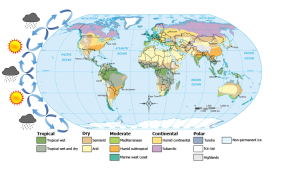
After rising and producing rain near the equator, the air masses move towards 30o latitude and sink back towards Earth as part of the Hadley convection cells. This air has lost most of its moisture after producing the equatorial rains, so the sinking air is dry, resulting in arid climates near 30o latitude in both hemispheres. Many of the major desert regions on Earth are located near 30o latitude, including much of Australia, the Middle East, and the Sahara Desert of Africa (Figure 6.3.1). The air also becomes compressed and heats up as it sinks, absorbing any moisture from the clouds and creating clear skies. Thus high pressure systems are associated with dry weather and clear skies. This cycle of high and low pressure regions continues with the Ferrel and Polar convection cells, leading to rain and the boreal forests at 60o latitude in the Northern Hemisphere (there are no corresponding large land masses at these latitudes in the Southern Hemisphere). At the poles, descending, dry air produces little precipitation, leading to the polar desert climate.
The elevation of the land also plays a role in precipitation and climactic characteristics. As moist air moves over land and encounters mountains it rises, expands, and cools because of the declining pressure and temperature. The cool air holds less water vapor, so condensation occurs and rain falls on the windward side of the mountains. As the air passes over the mountains to the leeward side, it is now dry air, and as it sinks the pressure increases, it heats back up, any moisture revaporizes, and it creates dry, deserts regions behind the mountains (Figure 6.3.2). This phenomenon is referred to as a rain shadow, and can be found in areas such as the Tibetan Plateau and Gobi Desert behind the Himalayas, Death Valley behind the Sierra Nevada mountains, and the dry San Joaquin Valley in California.

Rising and falling air are also responsible for more localized, short-term wind patterns in coastal areas. Due to the high heat capacity of water, land heats up and cools down about five times faster than water. During the day the sun heats up the land faster than it heats the water, setting up a convection cell of warmer rising air over the land and sinking cooler air over the water. This creates winds blowing from the water towards the land during the day and early evening; a sea breeze (Figure 6.3.3). The opposite occurs at night, when the land cools more quickly than the ocean. Now the ocean is warmer than the land, so air rises over the water and sinks over the land, creating a convection cell where winds blow from land towards the water. This is a land breeze, which blows at night and into the early morning (Figure 6.3.3).

The same phenomenon leads to seasonal climatic changes in many areas. During the winter the lower pressure is over the warmer ocean, and the high pressure is over the colder land, so winds blow from land to sea. In summer the land is warmer than the ocean, causing low pressure over the land and winds to blow from the ocean towards the land. The winds blowing from the ocean contain a lot of water vapor, and as the moist air passes over land and rises, it cools and condenses causing seasonal rains, such as the summer monsoons of southeast Asia (Figure 6.3.4).


Suborder Pinnipedia: seals, sea lions and walruses
- As a group, they are fairly young, having been around for only about 25 million years
- They consist of three families:
- Otariidae: sea lions
- Phocidae: true seals
- Odobenidae: walruses
Otariidae (sea lions) have:
- small, but obvious external ears
- long, flexible necks
- independent and mobile hind limbs that permit substantial movement on land
- nostrils that are located at the tip of the snout
- a dense and soft undercoat of fur protected by a layer of long and close guard hairs
- several layers of blubber for thermal protection
Phocidae (true seals) differ from sea lions in that they have:
- no external ears
- smooth, coarse hair with no underfur
- a hind limb geometry that restricts their ability to move on land
- fore flippers that are smaller than those of sea lions
Diving behavior and adaptations:
- can shut off their nostrils completely while diving
- can restrict the flow of blood to vital organs
- can store lots of oxygen
- routinely dive for 30 minutes
Odobenidae (Walruses):
- These creatures are limited to the Arctic waters of the Atlantic and Pacific
- Their hind limbs are similar to those of sea lions, but they have no external ear
- The most characteristic feature is the set of enlarged canine teeth – tusks
- They may weigh up to 4000 pounds
- They have air sacs in their cheeks that are used for support like a life preserver because they sleep in the water
- They also use their tusks to hoist themselves out of the water onto the ice
- They are becoming rare; estimates of their numbers world-wide are around 170,000 to 190,000 individuals
Suborder Fissipedia: sea otters
- These members of the weasel family are included in the list of marine mammals because they do live in the sea and are obviously mammals
- They have the distinction of having the most valuable fur of any animal – their dark brown underfur is extraordinarily dense and rich
- Trade in sea otter fur dates back at least to 1786, under the Spanish
- This became the most important industry of the California coast and most otters were gone by 1850
- In 1900, a small population was discovered near Bixby Creek on the central California coast
- Today, the otter's range has expanded along much of the Pacific coast of the U.S., especially since their protection under the Marine Mammal Protection Act of 1972
- They grow to lengths of about 5 ft and weights up to about 80 pounds
- They live for up to about 20 years
- They live by foraging for urchins, abalone, mussels, clams, crabs and other tidbits in relatively shallow water, rarely diving deeper than 60 ft
- They frequently consume their food in an attitude of leisure as they float gently on their backs
- They will use stones to smash open shellfish by placing them on their stomachs as they float on their backs
- They have very high metabolic rates and must consume up to 25% of their body weight each day in order to maintain an internal temperature of 100 degrees
- This works out to be about 140 to 190 calories per kilogram of body weight per day, compared to the average human who must consume about 32 calories per kilogram of body weight per day
- If a sea otter doesn't eat for a day, it will lose up to 10% of its body weight
- They live in the kelp forests and use the kelp as a tether while resting at the surface
- They help protect the kelp forest by eating the sea urchins that feed on the kelp
- They also use the kelp as protection from their enemies, including sharks and killer whales
- Making them a keystone species in this habitat
Order Sirenia: Sea Cows
- This group includes the manatees, which are found in the shallow bays and estuaries of North America and Africa, and the dugongs, which are found in the Indo-Pacific regions
- They're common in the winter in the warm-springs of Florida's west coast including the Crystal River
- These lovable rascals feed on water plants (especially the hyacinths in southern canals) – they're strictly vegetarians
- They show little fear of man and, as a result, many are killed or severely injured each year by boat propellers, as they have a habit of swimming just below the surface
- They form family groups and appear to show great care and affection for family members
The Sirenians were named after the Sirens, the mermaids that Ulysses encountered on his return from the Trojan wars – apparently they resembled mermaids to sailors who had been too long at sea or were drunk (or maybe both)...
Dr. Cristina Cardona
Major types of ocean pollution include:
* Petroleum
* Sewage
* Solid Waste
* Heavy metals and toxic chemical compounds.
Critical factors relating to ocean pollution:
*There are many ways these substance end up in the ocean ranging from intentional dumping, acts of ignorance, to impacts of disasters (natural and otherwise).
* The majority of pollutants come from land. According to NOAA studies, about 80 percent of marine pollution comes from sources on land.
* Man-made products become pollution when exposed to natural processes that move them through the natural environment. Materials like paper and metals typically decay, but plastic and glass can survive indefinitely. Plastic bags, nets, and fishing lines can trap, injure, and strangle marine creatures. Small plastic objects are consumed and block digestion. Inks, dyes, metals, and toxins from cigarette butts can poison sea life on all tropic levels.
Pollution and Pollutants
Pollutants comes in many forms and from many sources. The impact of pollutants depends on many factors: chemical properties, concentrations, what they react with, what they can convert into, and where and how they may become concentrated through both physical or biological processes. Some chemicals are purely toxic, poisoning organisms in small concentrations, loss of biomass, interfering with reproduction, growth, neurological and respiration function, and causing organ failure, and death. Some chemical pollutants make it more difficult for water to hold gases (such as O2 and CO2). Solids in silt or clay form can cover benthic animal, clog filter feeders, and block sunlight.
Some chemicals bio-amplify. Biologic amplification involves a toxin that does not metabolize and accumulates in an organism, and possibly increases in concentrations as it moves up the food chain. For example the banned insecticide DDT goes through biologic amplification as it is consumed in a contaminated ecosystem. DDT is an example of a chemical pollutant that goes through biologic amplification as it moves up the food chain (Figure 12.10).
Tropic Level in Food Chain |
DDT concentration |
Phytoplankton | 0.000003 ppm. |
Zooplankton | 0.04 ppm |
Small fish | 0.5 ppm |
Large fish | 2.0 ppm |
Birds | 25 ppm |
Figure 17.10. Biologic amplification is illustrated by DDT. Through political action it was finally banned in 1972 by the United States Environmental Protection Agency. DDT is discussed more below.
Point and Non-Point Sources of Pollution
All pollution has a source of origin. Point sources are visibly obvious sources. The U.S. Environmental Protection Agency (EPA) defines point source pollution as “any single identifiable source of pollution from which pollutants are discharged, such as a sewage outfall, a ship, or a smokestack.” Strict laws and regulations implemented in recent decades have helped reduce pollution from point sources in most developed countries (mostly because they are visibly obvious and can be linked directly to an original fiscally-responsible source). However, the major contributions to marine pollution come from non-point sources associated with runoff from land (Figures 17-11 and 17-12). Some ocean pollution starts as air pollution. Non-point sources include small sources (cars, trucks, boats, septic tanks, chimney smoke), and larger sources including agricultural wastes (pesticides, herbicides, fertilizer, and animal wastes) from farms, ranches, and harvesting forest areas. Runoff from industrial and urban areas (roads, parking lots, roofs), faulty managed industrial operations, and poorly-designed waste-water treatment facilities contribute pollutants and garbage to marine settings.
Pollution, and the secondary effects of pollution (causing harmful bacterial and algal blooms), can be very harmful to both wildlife and humans. About a third of shell-fish growing coastal waters are adversely impacted by pollution. Coastal waters and beaches are often unsafe for swimming after storm runoff.
Environmental Concerns with Petroleum Industry Activities
As discussed in previous chapters, most scientists point to fossil fuel consumption as a primary cause for global warming and associated climate change—a topic of greatest concern for the future fate of humanity and the quality and biodiversity of the world's physical environments. More discussion related to petroleum pollution is discussed below under Marine Pollution. The burning of fossil fuels (oil, gas, coal) are a primary factor impacting climate change. Although alternative energy sources are gradually reducing the demand for fossil fuels in the United States, the consumption worldwide is still gradually increasing..
Petroleum exploration, production, and consumption are the leading causes of pollution affecting the oceans. Petroleum includes crude oil, refined oil products, oil, gas, and tars (asphalt), petroleum derivatives (plastics, waxes, etc.), and greenhouse gases and toxins released by the production and burning of fossil fuels.
Sources of Carbon Dioxide (CO2) Emissions
Carbon dioxide entering Earth’s atmosphere comes from the oceans, soil, plants, animals and volcanoes. Greenhouse gases and pollution from the consumption of fossil fuels is now considered a major cause of climate change and changes in ocean chemistry detrimental to sea life. Climate change investigations show that although human sources of carbon dioxide are much smaller than natural emissions. However, human-source CO2 emission have been significantly increasing since the Industrial Revolution began in the 19th Century. Human activities are offsetting the balance between “sources” of emissions and “sinks” that remove CO2 from the atmosphere. Currently carbon dioxide emissions from fuel combustion include about 43% from coal, 36% is produced by oil, and 20% from natural gas. Destruction of natural “sinks” on land are cause by deforestation and degradation of organic-rich soils in developing agricultural regions which are also releasing large quantities of CO2 into the atmosphere.
Crude oil is a natural resource. Petroleum (gas, oil, and tar) form naturally in the Earth’s crust and are derived from sedimentary deposits, mostly from the continental margin regions of the world (both ancient and modern settings. Crude oil and gas forms from the slow biological and thermal decay of organic remains and residues buried in sediments. It typically takes many millions of years for organic-rich sediments deposited in coastal swamp regions, continental shelves, or the deep ocean to go through the processes to be converted to petroleum resources. The conversion involves: how much organic matter occurs in sediments, how much heat the sediments are exposed to, and for how long it is exposed. The more time organic matter is exposed to heat, the more it breaks down to by-products of gases, fluids (oil), and heavy carbon-rich residues (tar), (Figure 17.14). Most of these materials remained trapped in sediments, but gas and oil can migrate to underground reservoirs or escape to the surface.
Unrefined crude oil is generally biodegradable. Many microscopic organisms (chiefly bacteria) will consume crude oil, however, refine oil products are generally non-biodegradable and are more toxic to wildlife. Light, volatile components will gradually evaporate. Heavier, carbon-rich residues can take much longer. Crude oil breaks down faster with higher temperatures, so spills in cold-water settings take much longer to break down.
Tar balls are a common feature found on beaches around the world. It must be noted that roughly half of the tar balls found on beaches come from natural sources (oil seeps on the seafloor). The other half comes from human activity (pollution). Natural tar seeps are typically common in oil production regions. For instance, anyone visiting the beaches in and around Santa Barbara, California will note that their shoes will become covered with tar after walk on the beach. Although there is oil drilling and production operations both offshore and onshore in the region, the tar on the local beaches mostly comes from natural sources: seeps on the seafloor.
From Miracosta College, is shared under a not declared license and was authored, remixed, and/or curated by LibreTexts. Download this book for free at https://geo.libretexts.org/Bookshelves/Oceanography/Oceanography_101_(Miracosta)
Our modern understanding of tide formation stems from Isaac Newton's Law of Universal Gravitation, which states that any two objects have a gravitational attraction to each other. The magnitude of the force is proportional to the masses of the objects, and inversely proportional to the square of the distance between the objects, according to the equation in Figure 3.5.1.
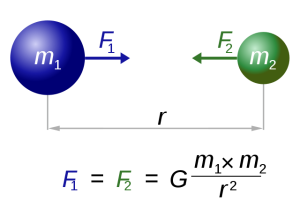
In the case of tides, there are a few other factors that modify this equation so that the distance (r) is cubed rather than squared, giving distance an even greater impact on tidal forces. But for our purposes, the important lesson is that the greater the masses of the objects, the greater the gravitational force, and the farther the objects are from each other, the weaker the force.
Such a gravitational force exists between the Earth and moon, attempting to pull them towards each other. Since the water covering Earth is fluid (unlike the solid land that is more resistant to tidal forces), this gravitational force pulls water towards the moon, creating a "bulge" of water on the side of the Earth facing the moon (Figure 3.5.2). This bulge always faces the moon, while the Earth rotates through it; the regions of Earth moving through the bulge experience a high tide, while those parts of the Earth away from the bulge experience a low tide.
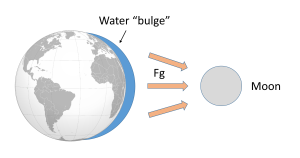
If the tides were this simple, everywhere on Earth would see one high tide per day, as there would only be a bulge of water on the side closest to the moon. However, if you have ever looked at tide charts, or lived near the ocean, you probably know that in most places there are two high tides and two low tides per day. Where is this second high tide "bulge" coming from?
The gravitational force between the Earth and moon might be expected to draw the two objects closer together, however, this is not happening. This is because the inward gravitational force is opposed by outward forces that keep the Earth and moon apart. The outward force is an intertial force created by the rotation of the Earth and moon. Contrary to popular belief, the moon is not simply rotating around the Earth; in fact, the Earth and moon are both rotating around each other. Imagine the Earth and moon as equal-sized objects revolving around a point at their center of mass. If both objects had the same mass, the center of rotation would be a point equidistant between the two objects. But since the mass of the Earth is 82 times greater than the mass of the moon, the center of revolution must be closer to the Earth. As an analogy, think about two people on a see-saw. If the people are of roughly equal size, they can sit on either end of the see-saw at it will rotate around a point at equal distance between them. But if the two people have very different masses, such as a large adult and a small child, the larger person must move closer to the pivot point for the see-saw to rotate effectively. In the same way, the center of rotation between the Earth and the moon (the barycenter) must be located closer to the Earth. In fact, the center of rotation lies within the Earth, about 1600 km below the surface. As the Earth and moon rotate around the barycenter, the moon travels much farther than the Earth, giving the impression that the moon is rotating around Earth (Figure 3.5.3).

The rotation of the Earth-moon system creates an outward inertial force, which balances the gravitational force to keep the two bodies in their orbits. The inertial force has the same magnitude everywhere on Earth, and is always directed away from the moon. Gravitational force, on the other hand, is always directed towards the moon, and is stronger on the side of the Earth closest to the moon. Figure 3.5.4 describes how these forces combine to create the tidal forces. At point O in the center of the Earth, the gravitational force (Fg) and the inertial force (Fr) are equal, and cancel each other out. On the side of Earth closest to the moon, the inward gravitational force (Fg) is greater than the outward inertial force (Fr); the net resulting force (A) is directed towards the moon, and creates a bulge of water on the side facing the moon. On the side of Earth opposite the moon, the outward inertial force is greater than the inward gravitational force; the net resulting force (C) is directed away from the moon, creating a water bulge directed away from the moon.
Now, as the Earth rotates through a 24 hour day, each region passes through two bulges, and experiences two high tides and two low tides per day. This represents Newton's Equilibrium Theory of Tides, where there are two high tides and two low tides per day, of similar heights, each six hours apart. But as with everything else in oceanography, reality is much more complex than this idealized situation.

Some of the additional complexity is because in addition to the moon, the sun also exerts tide-affecting forces on Earth. The solar gravitational and inertial forces arise for the same reasons described above for the moon, but the magnitudes of the forces are different. The sun is 27 million times more massive than the moon, but it is 387 times farther away from the Earth. Despite its larger mass, because the sun is so much farther away than the moon, the sun's gravitational forces are only about half as strong as the moon's (remember that distance is cubed in the gravity equation). The sun thus creates its own, smaller water bulges, independent of the moon's, that contribute to the creation of tides.
When the sun, Earth and moon are aligned, as occurs during new and full moons, the solar and lunar bulges are also aligned, and add to each other (constructive interference; see section 4.2) creating an especially high tidal range; high high tides and low low tides (Figure 3.5.5). This period of maximum tidal range is called a spring tide, and they occur every two weeks.

When the sun, Earth and moon are at 90o to each other, the solar and lunar bulges are out of phase, and cancel each other out (destructive interference). Now the tidal range is small, with low high tides and high low tides (Figure 3.5.6). These are neap tides, and occur every two weeks, when the moon is in its 1/4 and 3/4 phases (Figure 3.5.7).


By Paul Webb, used under a CC-BY 4.0 international license. Download this book for free at https://rwu.pressbooks.pub/webboceanography/front-matter/preface/
-
- a streamlined body shape for more efficient movement through the water
- a high metabolic rate that generates a lot of heat and layers of fat and, in some cases, fur, to conserve this heat
- modifications to their respiratory system to collect and retain large volumes of oxygen to allow deep and repetitive dives
- osmotic adaptations that free them from any requirement for fresh water
- https://www.noaa.gov/education/resource-collections/marine-life/marine-mammals
Order Cetacea: the whales
- Whales also have special adaptations, such as:
- A thick layer of blubber
- Their nostrils have migrated to the top of the head so that the animal doesn't have to come completely out of the water to breathe
- Large, deeply convoluted brains
- A special skin that "gives" to dampen out irregularities in water flow
- Bronchial cartilage that supports their lungs against pressure during deep dives
- Their blood is especially rich in hemoglobin to store more oxygen for diving
- They can slow their heartbeat while diving
- The blood supply can be reduced to all but the vital organs while diving, to conserve oxygen
Whale groups come in two 'flavors' (sub-Orders) – Odontocetes (toothed whales) and Mysticetes (baleen whales)
- Sperm whales are the largest of the toothed whales, reaching lengths up to 60 ft
- It also possesses the largest brain ever to have evolved on Earth
- They can dive to greater depths than any other air-breathing animal – to 3000 ft – and can stay down for over an hour
- Their head is filled with up to a ton of clear oil, presumably used to focus sound waves passing to their prey and back
Mysticetes (baleen whales): Also known as baleen whales, the Mysticetes are considered to be more highly evolved than the Odontocetes. Baleen is a bristly, bush, fibrous substance set in the jaws in overlapping plates; it looks like a gigantic comb.
- They feed on small planktonic arthropods (krill)
- Baleen is a straining mechanism
- The whale takes in a big gulp of water, closes its jaws, raises its tongue to expel the water, and the food is caught on the surface of the baleen
- The food is swallowed after being licked from the baleen by the whale's giant tongue
- Baleen whales include the blue, humpback and gray, among others

- The blue whale is probably the largest animal ever to have lived on Earth
- They're usually described as up to about 100 feet in length and up to about 200 tons
- this compares to three railroad cars in length and about 1600 people in weight
- Blue whales feed on krill, which are tiny shrimp-like crustaceans
- A mature blue whale consumes about 4 tons of krill per day when it's feeding
- For a 7 to 8 month period; it fasts when it is in tropical waters
- From December through April, the blue whale gorges on krill around the Antarctic
Humpback whale
- Humpbacks are stocky and seldom exceed 50 ft in length
- They're generally black above and white below, with extremely long, wing-like flippers
- They've become known for their "song", which are sounds with definite patterns and sequences
- All the whales in a given population sing the same song, but the song changes every year
- Humpbacks are also very acrobatic, at times leaping completely out of the water
- It is estimated that there are no more than 10,000 humpbacks surviving today
Gray whale
- The Gray whale is the only large whale with a heavily mottled appearance and a knobby ridge down the back
- Its 'natural' color is dark gray or black, but it's covered by a profusion of spots, scars, patches, and clusters of barnacles, which gives it a mottled, grayish appearance
- The Gray whale makes the longest migration of any mammal, an annual round-trip of some 10,000 miles from the Bering and Chukchi seas in the high Arctic to the warm lagoons of Baja California
- They were easily caught by whalers and almost became extinct in the 1940's
- Given full protection in 1946, they've made a successful comeback and their population, estimated at about 21,000, is now believed to be inline with the carrying capacity of their range
- The Gray whale is the most heavily parasitized of all cetaceans – playing host to three species of lice, some over an inch long – in one case, more than 100,000 of one species of louse were removed from a single whale
Written by Dr. Cristina Cardona.
Sea salts are generated from from seawater evaporated in ponds. About 1/3 of all table salt (NaCl) in the world comes from evaporation seawater. Other economically significant mineral derivative from seawater evaporation operations include gypsum and salts of potassium, magnesium, iodine, and bromine.
Iron-manganese nodules are hydrogenous rocks that precipitated from seawater. They form very slowing (taking millions of years to form fist-size nodules, Figure 12.3.1). However, large regions of abyssal plains in the deep ocean are covered with them, particularly in the southern Pacific Ocean.. Samples collected show that they are rich in iron, manganese, copper, nickel, and cobalt. Mining manganese nodules from the deep sea bed is not considered economically feasible at this time. Similarly, deposits around deep sea vents (black smokers) are also rich in valuable metals but mining them is not economically feasible. However, ancient back smoker and manganese nodule deposits have been discovered and mined on land.
Figure 12.3.1. Iron-manganese nodules on the seafloor on an abyssal plain in the South Pacific Ocean.
From Miracosta College, is shared under a not declared license and was authored, remixed, and/or curated by LibreTexts. Download this book for free at https://geo.libretexts.org/Bookshelves/Oceanography/Oceanography_101_(Miracosta)
Seafood (fish, crustaceans, and mollusks) is perhaps the most valuable biological resource. An estimated 86.6 million tons/yr., or 170,000 million pounds, of seafood are harvested around the world each year. Most seafood is harvested from temperate latitudes.
Seafood consumption has been gradually increasing worldwide, meanwhile fish and other seafood supplies have been diminishing, largely due to overfishing in poor, developing regions of the world. China alone consumes about 1/3 of all seafood harvested each year.
Aquaculture is gradually replacing traditional "capture" deep sea fishing. In the early 1970s only about 1 percent of seafood came from fish farms (aquaculture). Today, many kinds of seafood are farm raised - most abundant are freshwater-trout, catfish, and crayfish, but marine aquaculture is increasing, primarily salmon, oysters, mussels, shrimp, crabs, lobster, and other crustaceans. Aquaculture now account for more than 80% of the seafood in China, and nearly about 30% consumed by the rest of the world. Most varieties of commercial fish are now farm raised or captured in a managed and (hopefully) sustainable manner (Figure 12.4.1). Capture fishing has become increasingly infeasible in many parts of the world where many ocean fish stocks have been depleted. Traditional hook-and-net style fishing has many environmental problems. Drag nets scour the seafloor, destroying benthic communities, and large nets capture and kill unintended sea creatures including sea turtles, marine mammals, and many other endangered species. Lost nets, fishing lines, and trash from fishing ships are a major source of trash in marine pollution. Many advances are being made in marine aquaculture, however, it too can have environmental consequences including generating large quantities of fish wastes, interfering with native species populations (including spreading diseases), and taking space away from natural benthic communities.
![]() |
|
Figure 12.4.1 Most sushi prepared in the United States are from farm-raise species. |
Commercial whaling in the 19th and 20th centuries had a devastating impact on many marine mammal species. Whales were harvested for meat, oil, and for fertilizer. Whaling operations peaked in the 1950-60's with approximately 60,000 whales a year. As a result, whale populations crashed. 8 out of 11 species are now considered "commercially extinct." Whale population estimates are that 4.4 million commercial whales were in the world resource population in 1900, but only about 1 million are estimated to exist today. Large-scale commercial whaling operations ceased in 1987 worldwide with exception of small operations still being conducted by Japan, Norway, and some coastal North American tribes.
Marine plants are being farm raised for food and other purposes. For example, extracts from kelp is used as a clarifier in beer and to form emulsions in salad dressings, inks and paints.
From Miracosta College, is shared under a not declared license and was authored, remixed, and/or curated by LibreTexts. Download this book for free at https://geo.libretexts.org/Bookshelves/Oceanography/Oceanography_101_(Miracosta)
Pfeiffer Beach, California 2023 (Photo credit: Cristina Cardona)
This book is a collection of Open Educational Resources adapted and edited by Dr. Cristina Cardona, Associate Professor of Physical Sciences.
Contributing authors:
- Cristina Cardona, Community College of Baltimore County Essex
- Paul Webb, Roger Williams University
- Miracosta College faculty
- University of California, Davis (Hill et al.)
- University of California, Davis (Keddy)
- Steven Earle