2 1.2 Continental Margins
Continental margins refer to the region of transition from the land to the deep seafloor, i.e. between continental and oceanic crust. In an active continental margin, the boundary between the continent and the ocean is also a tectonic plate boundary, so there is a lot of geological activity around the margin. The west coast of the United States is an example of an active margin, where the coastline corresponds with the boundary between the Pacific and North America Plates. A passive continental margin occurs where the transition from land to sea is not associated with a plate boundary. The east coast of the United States is a good example; the plate boundary is located along the mid Atlantic ridge, far from the coast. Passive margins are less geologically active. Figure 1.2.1 shows an idealized passive margin. When examining this figure, and others like it, note that there is significant vertical exaggeration; the depth scale covers approximately 5000 m, while the horizontal scale extends around 300 km. This makes the features look much steeper than they actually are. The bar at the bottom of Figure 1.2.1 shows what a passive margin would look like without this exaggeration; there is a much more gradual transition to depth.
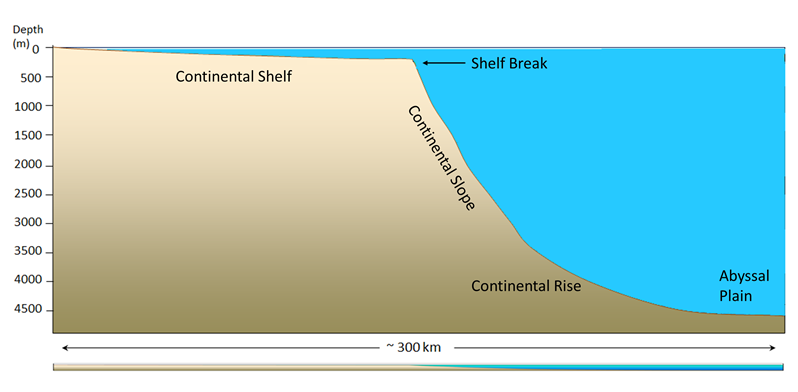
The continental shelf is the shallow, flooded edge of the continent. Geologically the shelf is still part of the continental crust, but it is often overlaid with marine sediments. On average, the shelf extends about 80 km from the coast; some margins have very little shelf, while the Siberian Shelf in the Arctic extends roughly 1500 km. The depth of the shelf generally remains below about 150 m, and the floor of the shelf is fairly flat. The flat topography is the result of changes in sea level; throughout history the shelves have been both submerged and exposed, and as sea level rose and fell, wave action, ice sheets, and other erosional processes smoothed out the shelf surface. Wave action and the movement of sediments over the shelf have continued this smoothing process. Continental shelves only make up about 6% of the ocean’s surface area, but they are biologically one of the richest parts of the ocean; their shallow depth prevents nutrients from sinking out, and their proximity to the coast provides significant nutrient input. The continental shelf ends at the shelf break, which is the point where the angle of the seafloor begins to get steeper. The shelf break averages about 135 m deep.
After the shelf break, the seafloor takes on a steeper angle (about 4o) as it descends to the deep ocean. This steeper portion of the margin is the continental slope, and it extends from the shelf break down to 3000-5000m. In some parts of the ocean, large submarine canyons have been carved into the continental slope; for example, Monterey Canyon in Monterey Bay, California, is a submarine canyon similar in size to the Grand Canyon! These canyons may be carved out by turbidity currents, which are essentially landslides of sediment, rocks, and other debris down the face of the slope.
At the bottom of the slope is the continental rise. This area represents where the continental crust meets the oceanic crust, as the slope begins to level off to become the deep ocean floor. The rise consists of a thick layer of accumulated sediment coming from the continent, so it is difficult to tell where the slope ends and the rise begins.
After the rise comes the abyssal plain, or the deep ocean floor, lying between 4500 – 6000 m. The abyssal plain includes most of the ocean floor, and is the flattest region on Earth. It is flat due to millions of years of sediment accumulation on the bottom, which buries many bottom features (Figure 1.2.2).
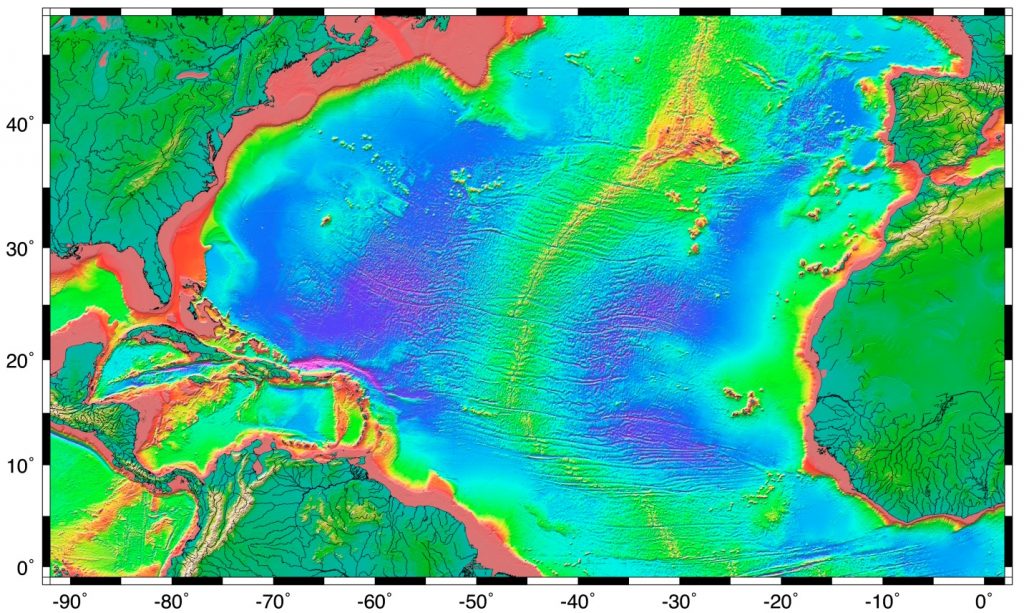
Passive margins, as described above, have wide shelves, gentle slopes, and a well-developed rise. Since passive margins are not plate boundaries, they experience long periods of relative stability which can lead to the development of these features. Active margins have similar features to passive margins, but the plate boundary affects the properties of the features. Active margins, like the Pacific coast of North America, have narrower shelves, steeper slopes, and little to no rise, particularly in convergent boundaries. Trenches associated with subduction zones act as sediment traps, preventing the accumulation of a continental rise, and keeping sediments off of the abyssal plains.
Marine Reptiles
Marine reptiles are cold blooded (ectothermic), meaning that their internal temperature is regulated by their surroundings and is not constant. They have scales that cover their bodies, they reproduce out of the water, and they evolved from amphibians. Today's marine reptiles include turtles, sea snakes, iguanas, and marine crocodiles.
Sea Turtles:
- 5 widely distributed tropical and subtropical species:
- Green, Hawksbill, Ridley, Leatherback and Loggerback
- All have large limbs and non-retractable heads
- All are excellent swimmers: the front limbs are flattened to act as oars, while the hind limbs work as rudders
- All grow to considerable size: the Atlantic Leatherback is the largest, growing to more than 1500 lbs and 11.5 ft long
- All are under threat of extinction
- The Green turtle is known for its long migrations, often of more than a thousand miles, between its feeding grounds and breeding areas
- Some use smell and vision, wave patterns, the angle of the sun and even celestial navigation to find a beach site close to where they were hatched
- if they survived there, then their offspring is more likely to survive
- https://www.seeturtles.org/sea-turtle-facts
Sea snakes:
- There are more than 50 species, which represent the most recently evolved group of marine reptiles
- They're found primarily in the warm oceans of the Indo-Pacific, but some can be found in the Atlantic (as seen in my photo)
- They grow to lengths of 4 to 10 feet
- Their bodies are flattened for swimming
- They breathe air, but have valves on their noses and can dive for crustaceans or shellfish
- They're truly marine except for one species that lays eggs on land – most give live birth at sea
- They also feed on small fish
- by catching and holding them in their jaws with their small teeth until their venom seeps into the wounds and kills the prey
- Their venom is among the most active of all known biological poisons
- the physiological effects are similar to those of cobra venom, only several times more toxic
- Fortunately for humans, they lack fangs to inject the venom and they are not terribly aggressive
- However, several people a year die from the bites of sea snakes, mostly fishermen who accidentally get bitten while removing them from their nets

Marine Iguanas:
- Found in the Galapagos Islands
- They're large, heavy and sluggish on land, but much more graceful in the sea
- They eat encrusting algae that they scrape from rocks with their spade-like teeth

Marine Crocodiles:
- These are occasional seafarers
- And are found mostly in the Indo-Pacific area from India to Australia
- They may grow to 30 ft in length
- They may be endangered because of predation by humans
Written by Dr. Cristina Cardona.
In addition to fish, the ocean is also home to a variety of birds. These birds are referred to as seabirds because they spend a considerable amount of time around the sea. Many seabirds spend their entire lives in the ocean, only coming to land to reproduce. Some seabirds dive and swim below the surface, such as penguins, whose wings are modified into flippers, or cormorants, whose webbed feet act as paddles. Others stay at the surface, only hunting in the uppermost meter of the water (Keddy). Please go to this website to read more about seabirds: https://ca.audubon.org/what-s-seabird
Birds evolved from reptilian ancestors
Birds split from the main reptile branch about 150 million years ago with an intermediate form known as Archaeopteryx, which was about the size of a crow. They have reptilian characteristics, such as their feet and lower legs are covered with scales and terminate in claws, their reproductive physiology is basically reptilian (laying eggs), their feathers are believed to have been derived from scales, and have many other developmental and structural similarities with reptiles. Yet, unlike reptiles, birds are warm-blooded (endothermic) and maintain a constant internal body temperature.
Marine birds
Marine birds tend to be larger and stronger than their land counterparts. They have a lightweight, low-density body structure with hollow bones. Their wings are long, pointed and cupped underneath to support a large and muscular organism aloft for long periods of time.
They do not drink freshwater. Instead, they have salt glands over their eyes that remove the salt, thus permitting them to drink seawater, freeing them from dependence on freshwater from the land. The salty fluid is expelled through their bills.
The albatross
- This is one of the largest of the oceanic birds, with a wingspan of up to 12 feet
- They are the best gliders in the world – may remain aloft for months at a time, taking advantage of the west wind drift (winds that blow completely around Antarctica)
Pelicans
- This prehistoric-looking bird feeds by diving on its prey and entrapping it in its large gular pouch
- It has air sacs in its shoulders that absorb the impact with the water
Arctic Terns
- This bird feeds on small fish swimming near the surface that it catches by diving. It may even swim below the surface after them
- It lives at high latitudes and breeds on all far north rocky coasts
- However, it dislikes cold weather, so makes the longest migration of any animal, 15,000 miles each way
Penguins
- Penguins are found only in the southern hemisphere
- They are flightless birds that "fly" through the water after fish, squid and krill
- They slow their heart rate
- Have subcutaneous fat layers for insulation
- Have heavy plumage for insulation
- Their bodies are streamlined
- They have heavy feet for paddling and kicking against the water
- Their blood is shunted to the heart and brain and the extremities are deprived of blood, in order to slow heat loss
- These apply to most diving birds
Emperor Penguin: These are the largest diving birds in the world, up to 4 ft in height
- They're the best bird divers in the world, can routinely dive for 5-10 minutes (most bird dives last 30 seconds to a minute) and at best can stay down for 15-18 minutes, diving to over 1000 feet
- They eat small fish and krill – penguin populations have increased as a result of the killing off of the great whales – more krill is available as food for penguins
Penguin Adaptations
- They fluff their feathers to create trapped air in a dead space
- They have long feathers
- They rock back and forth on their heels on the ice to limit the area in contact with the ice and slow heat loss
- They can reduce blood flow to and heat loss from their wings and feet
- They exhibit "huddle" behavior – gathering in large groups and constantly changing position so that each gets a turn in the center, where it's warmest
The first paragraph, by Keddy (University of California, Davis), is shared under a not declared license and was authored, remixed, and/or curated by LibreTexts. Download this book for free at https://geo.libretexts.org/Courses/Diablo_Valley_College/OCEAN-101%3A_Fundamentals_of_Oceanography_(Keddy)
The rest was written by Dr. Cristina Cardona.
Sea salts are generated from from seawater evaporated in ponds. About 1/3 of all table salt (NaCl) in the world comes from evaporation seawater. Other economically significant mineral derivative from seawater evaporation operations include gypsum and salts of potassium, magnesium, iodine, and bromine.
Iron-manganese nodules are hydrogenous rocks that precipitated from seawater. They form very slowing (taking millions of years to form fist-size nodules, Figure 12.3.1). However, large regions of abyssal plains in the deep ocean are covered with them, particularly in the southern Pacific Ocean.. Samples collected show that they are rich in iron, manganese, copper, nickel, and cobalt. Mining manganese nodules from the deep sea bed is not considered economically feasible at this time. Similarly, deposits around deep sea vents (black smokers) are also rich in valuable metals but mining them is not economically feasible. However, ancient back smoker and manganese nodule deposits have been discovered and mined on land.
Figure 12.3.1. Iron-manganese nodules on the seafloor on an abyssal plain in the South Pacific Ocean.
From Miracosta College, is shared under a not declared license and was authored, remixed, and/or curated by LibreTexts. Download this book for free at https://geo.libretexts.org/Bookshelves/Oceanography/Oceanography_101_(Miracosta)
The Equilibrium Theory of tides predicts that each day there will be two high and two low tides, each one occurring at the same time day after day, with each pair producing tides of similar heights. While this view provides a basic explanation for the primary forces that generate the tides, it does not take into account such variables as the effects of the continents, the depth of the water, and many other factors. In all, there are almost 400 variables that must be incorporated into predicting the tides! The Dynamic Theory of tides takes these other factors into account, and shows that the tides are much more complicated and variable from place to place than the Equilibrium Theory would suggest. For example, some areas receive only one high and one low tide per day (see section 3.7). Furthermore, the tidal range varies greatly across the globe; in the Mediterranean Sea, there can be a difference of only 10 cm between high and low tides, while the Bay of Fundy in Canada experiences a tidal range of up to 17m (56 ft) every day (Figure 3.6.1).
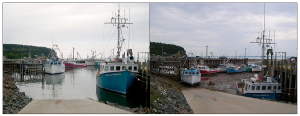
Examination of any tide chart will show that the tides don’t occur at same time each day; in fact, each tidal peak occurs about 50 minutes later than it did in the previous day. This is due to the orbit of the moon around the Earth. Imagine a high tide that occurs at a particular location (X) at 1:00 pm (Figure 3.6.2). The high tide occurs as location X moves through the bulge of water facing the moon. It will take the Earth 24 hours to complete one revolution, to bring location X back to site of the water bulge that caused that high tide. However, during those 24 hours, the moon has also moved as it orbits the Earth, so the high tide bulge has moved beyond its original location. The Earth thus has to rotate an additional distance for location X to reach the bulge and experience that same high tide. Because it takes the moon about 28 days to orbit the Earth, the moon gets "ahead" of the Earth's rotation by about 50 minutes per day. Therefore, it takes location X 24 hours and 50 minutes to rotate through the same tidal bulge, and as a result, the tidal peaks occur about 50 minutes later each day. In our example, an afternoon high tide at 1:00 pm on one day would be followed by a high tide at about 1:50 pm the following day. This 24 hour and 50 minute cycle is referred to as a tidal day.
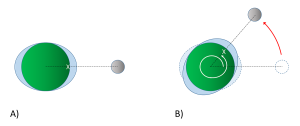
The motion of the moon impacts the tidal cycles in other ways. As the moon orbits the Earth, its orbital plane is at an angle relative to the rotational plane of Earth. This angle, or declination, means than the moon fluctuates between an angle of 28.5o north of the equator, to 28.5o south of the equator roughly every two weeks (the cycle from maximum to minimum and back takes about 27 days). Figure 3.6.3 illustrates a case where the moon is at its maximum declination 28.5o north of the equator, creating its corresponding tidal maxima. A point on the Earth at the latitude indicated by the red line would experience two high tides as it rotated through 24 hours, at points A and B. But the two high tides would not be of equal heights; the high tide at A would be higher than the high tide at B. This helps create a mixed semi-diurnal tide; two high tides of different heights per day (see section 3.7).
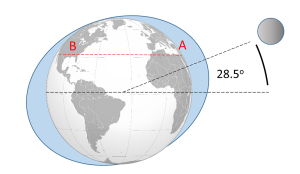
Finally, the continents and the bottom topography of the oceans have an impact on the tides that are experienced in an area. Because the tides are essentially waves with extremely long wavelengths extending halfway across the Earth, they behave as shallow water waves, and they are influenced and refracted by the bottom contours, leading to regional tidal variations. When the tidal crests encounter land, they are are reflected, and the wave moves back out to sea, theoretically until it encounters another continent on the opposite side of the ocean basin. The crest is once again reflected, and the water oscillates back and forth as a standing wave across the ocean basin. However, because of the scale over which these tidal waves move, we must take into account the influence of the Coriolis Effect. As the tidal crest is reflected back across the ocean basin, its path is deflected by the Coriolis force; to the right in the Northern Hemisphere, and to the left in the Southern Hemisphere. Using the Northern Hemisphere as an example, imagine a tidal crest that has reached land on the western side of an ocean basin. It would have a tendency to be reflected and move across the basin towards the east. But the Coriolis force deflects the movement to the right, causing the crest to instead head south. When the crest hits land in the south, it would now tend to reflect towards the north, but once again the Coriolis deflection to the right kicks in, and the wave instead moves to the east. From the east the reflected wave is deflected to the north, and so on. The result of all of this is that instead of a simple standing wave moving back and forth across the ocean, the tidal crest follows a circular pattern around the ocean basin, counterclockwise in the Northern Hemisphere and clockwise in the Southern Hemisphere. This is analogous to shaking a pan full of water in a circular manner, and watching the water follow a similar circular path as it sloshes around inside. This large scale circular rotation pattern of tides is called amphidromic circulation (Figure 3.6.4). The rotation occurs around a central amphidromic point or node, that shows little tidal variation, while the largest tidal ranges occur on the edges of the circulation pattern. In Figure 3.6.4 the amphidromic points are indicated by the dark blue areas where the white lines converge, like spokes from a bicycle wheel, and the dark red and brown areas show the regions of maximum tidal heights. The tidal maxima will rotate around the amphidromic points, taking about 12 hours for a complete rotation, leading to two high and two low tides per day in many places. If a tidal maximum is occurring along one of the white lines in Figure 3.6.4 at a certain time in the Northern Hemisphere, one hour later that high tide will have moved to the white line to the left (counterclockwise), and so on until it completes a rotation. In the Southern Hemisphere, the tide will move to the line to the right for clockwise rotation.
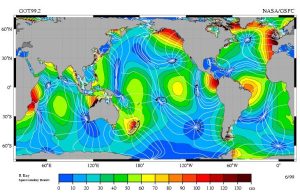
The result of all of these variables is that the tides will not always occur twice each day, at the same time and with equal heights as the Equilibrium Theory of tides may suggest. Instead, each region of the oceans has a unique set of factors that contribute to the types of tides it will experience. The major types of tides are discussed in the next section.
By Paul Webb, used under a CC-BY 4.0 international license. Download this book for free at https://rwu.pressbooks.pub/webboceanography/front-matter/preface/
-
- a streamlined body shape for more efficient movement through the water
- a high metabolic rate that generates a lot of heat and layers of fat and, in some cases, fur, to conserve this heat
- modifications to their respiratory system to collect and retain large volumes of oxygen to allow deep and repetitive dives
- osmotic adaptations that free them from any requirement for fresh water
- https://www.noaa.gov/education/resource-collections/marine-life/marine-mammals
Order Cetacea: the whales
- Whales also have special adaptations, such as:
- A thick layer of blubber
- Their nostrils have migrated to the top of the head so that the animal doesn't have to come completely out of the water to breathe
- Large, deeply convoluted brains
- A special skin that "gives" to dampen out irregularities in water flow
- Bronchial cartilage that supports their lungs against pressure during deep dives
- Their blood is especially rich in hemoglobin to store more oxygen for diving
- They can slow their heartbeat while diving
- The blood supply can be reduced to all but the vital organs while diving, to conserve oxygen
Whale groups come in two 'flavors' (sub-Orders) – Odontocetes (toothed whales) and Mysticetes (baleen whales)
- Sperm whales are the largest of the toothed whales, reaching lengths up to 60 ft
- It also possesses the largest brain ever to have evolved on Earth
- They can dive to greater depths than any other air-breathing animal – to 3000 ft – and can stay down for over an hour
- Their head is filled with up to a ton of clear oil, presumably used to focus sound waves passing to their prey and back
Mysticetes (baleen whales): Also known as baleen whales, the Mysticetes are considered to be more highly evolved than the Odontocetes. Baleen is a bristly, bush, fibrous substance set in the jaws in overlapping plates; it looks like a gigantic comb.
- They feed on small planktonic arthropods (krill)
- Baleen is a straining mechanism
- The whale takes in a big gulp of water, closes its jaws, raises its tongue to expel the water, and the food is caught on the surface of the baleen
- The food is swallowed after being licked from the baleen by the whale's giant tongue
- Baleen whales include the blue, humpback and gray, among others

- The blue whale is probably the largest animal ever to have lived on Earth
- They're usually described as up to about 100 feet in length and up to about 200 tons
- this compares to three railroad cars in length and about 1600 people in weight
- Blue whales feed on krill, which are tiny shrimp-like crustaceans
- A mature blue whale consumes about 4 tons of krill per day when it's feeding
- For a 7 to 8 month period; it fasts when it is in tropical waters
- From December through April, the blue whale gorges on krill around the Antarctic
Humpback whale
- Humpbacks are stocky and seldom exceed 50 ft in length
- They're generally black above and white below, with extremely long, wing-like flippers
- They've become known for their "song", which are sounds with definite patterns and sequences
- All the whales in a given population sing the same song, but the song changes every year
- Humpbacks are also very acrobatic, at times leaping completely out of the water
- It is estimated that there are no more than 10,000 humpbacks surviving today
Gray whale
- The Gray whale is the only large whale with a heavily mottled appearance and a knobby ridge down the back
- Its 'natural' color is dark gray or black, but it's covered by a profusion of spots, scars, patches, and clusters of barnacles, which gives it a mottled, grayish appearance
- The Gray whale makes the longest migration of any mammal, an annual round-trip of some 10,000 miles from the Bering and Chukchi seas in the high Arctic to the warm lagoons of Baja California
- They were easily caught by whalers and almost became extinct in the 1940's
- Given full protection in 1946, they've made a successful comeback and their population, estimated at about 21,000, is now believed to be inline with the carrying capacity of their range
- The Gray whale is the most heavily parasitized of all cetaceans – playing host to three species of lice, some over an inch long – in one case, more than 100,000 of one species of louse were removed from a single whale
Written by Dr. Cristina Cardona.
Tsunamis loom large in popular culture, but there are a number of misconceptions about these large waves. First, tsunamis have nothing to do with the tides, so it is a misnomer to refer to them as "tidal waves." There are actual tidal waves (see section 3.5), but they are not related to tsunamis. Second, the giant, curling wave that is taller than skyscrapers and destroys cities in science fiction movies is also a fabrication, as tsunamis do not behave that way, as described below.
Tsunamis are large waves that are usually the result of seismic activity, such as the rising or falling of the seafloor due to earthquakes, although volcanic activity and landslides can also cause tsunamis in the form of splash waves (see section 3.1). As the seafloor rises or falls, so does the water column above it, creating waves. Only vertical seismic disturbances cause tsunamis, not horizontal movements. These vertical seafloor movements are usually less than 10 m high, so the resulting wave will be of an equal or lesser height at sea. While the tsunamis have a relatively small height at the point of origin, they have very long wavelengths (100-200 km). Because of the long wavelength, they behave as shallow water waves throughout the entire ocean; the depth of the ocean is always shallower than half of their wavelength. As shallow water waves, their speed depends on water depth, but they can still travel at speeds over 750 km/hr (Figure 3.4.1)!
When tsunamis approach land, they behave just like any other wave; as the depth becomes shallower, the waves slow down and the wave height begins to increase. However, contrary to popular belief, tsunamis do not arrive on shore as giant, cresting waves. Since their wavelength is so long, it is impossible for their height to ever exceed 1/7 of their wavelength, so the waves don’t actually curl or break. Instead, they usually hit the shore as sudden surges of water causing a very rapid increase in sea level, like that of an enormous rise in tide. It may take several minutes for the wave to pass, during which time sea level can rise to 40 m higher than usual.
Large tsunamis occur every 2-3 years, with very large, damaging events happening every 15-20 years. The most devastating tsunami in terms of loss of life resulted from a magnitude 9 earthquake in Indonesia in 2004 (Figure 3.4.2), which created waves up to 33 m tall and left about 230,000 people dead in Indonesia, Thailand, and Sri Lanka. In 2011 a 9.0 magnitude earthquake in Japan triggered a tsunami up to 40.5 m high, which resulted in over 18,000 deaths. This earthquake also caused the Fukishima nuclear accident, and moved Japan about 8 inches closer to the U.S.

By Paul Webb, used under a CC-BY 4.0 international license. Download this book for free at https://rwu.pressbooks.pub/webboceanography/front-matter/preface/
Seafood (fish, crustaceans, and mollusks) is perhaps the most valuable biological resource. An estimated 86.6 million tons/yr., or 170,000 million pounds, of seafood are harvested around the world each year. Most seafood is harvested from temperate latitudes.
Seafood consumption has been gradually increasing worldwide, meanwhile fish and other seafood supplies have been diminishing, largely due to overfishing in poor, developing regions of the world. China alone consumes about 1/3 of all seafood harvested each year.
Aquaculture is gradually replacing traditional "capture" deep sea fishing. In the early 1970s only about 1 percent of seafood came from fish farms (aquaculture). Today, many kinds of seafood are farm raised - most abundant are freshwater-trout, catfish, and crayfish, but marine aquaculture is increasing, primarily salmon, oysters, mussels, shrimp, crabs, lobster, and other crustaceans. Aquaculture now account for more than 80% of the seafood in China, and nearly about 30% consumed by the rest of the world. Most varieties of commercial fish are now farm raised or captured in a managed and (hopefully) sustainable manner (Figure 12.4.1). Capture fishing has become increasingly infeasible in many parts of the world where many ocean fish stocks have been depleted. Traditional hook-and-net style fishing has many environmental problems. Drag nets scour the seafloor, destroying benthic communities, and large nets capture and kill unintended sea creatures including sea turtles, marine mammals, and many other endangered species. Lost nets, fishing lines, and trash from fishing ships are a major source of trash in marine pollution. Many advances are being made in marine aquaculture, however, it too can have environmental consequences including generating large quantities of fish wastes, interfering with native species populations (including spreading diseases), and taking space away from natural benthic communities.
![]() |
|
Figure 12.4.1 Most sushi prepared in the United States are from farm-raise species. |
Commercial whaling in the 19th and 20th centuries had a devastating impact on many marine mammal species. Whales were harvested for meat, oil, and for fertilizer. Whaling operations peaked in the 1950-60's with approximately 60,000 whales a year. As a result, whale populations crashed. 8 out of 11 species are now considered "commercially extinct." Whale population estimates are that 4.4 million commercial whales were in the world resource population in 1900, but only about 1 million are estimated to exist today. Large-scale commercial whaling operations ceased in 1987 worldwide with exception of small operations still being conducted by Japan, Norway, and some coastal North American tribes.
Marine plants are being farm raised for food and other purposes. For example, extracts from kelp is used as a clarifier in beer and to form emulsions in salad dressings, inks and paints.
From Miracosta College, is shared under a not declared license and was authored, remixed, and/or curated by LibreTexts. Download this book for free at https://geo.libretexts.org/Bookshelves/Oceanography/Oceanography_101_(Miracosta)