Distribution
Open Resources for Nursing (Open RN)
Distribution
The second stage of pharmacokinetics is the process known as distribution. Distribution is the process by which a drug is dispersed throughout the body’s blood and tissues. After a drug enters into systemic circulation by absorption or direct administration, it will pass from vascular spaces to tissues where a drug-receptor interaction will occur, creating the effect of the drug.
Drugs are designed to primarily cause one effect, meaning they bind more strongly to one specific receptor site and predictably cause or block an action. However, side effects and adverse effects can occur when the drug binds to other sites in addition to the target tissue, causing an unintended action. These side effects can range from tolerable to unacceptable and can result in the discontinuation of the medication. For example, a person might take the pain reliever ibuprofen (Advil) to treat a sore leg muscle, and the pain may be subsequently relieved, but there may also be stomach irritation as a side effect.
The distribution of a drug throughout the body is dependent on many body-related factors such as blood flow, tissue differences, plasma protein-binding, the blood-brain barrier, and the placental barrier.
Blood Flow
The circulatory system transports medications throughout the body in the bloodstream. Many factors can affect the blood flow and delivery of medication, such as decreased blood flow (due to dehydration), blocked vessels (due to atherosclerosis), constricted vessels (due to uncontrolled hypertension), or weakened pumping by the heart muscle (due to heart failure). As an example, when administering an antibiotic to a client with diabetes who has an infected toe, it may be difficult for the antibiotic to move through the blood vessels all the way to the area of the toe that is infected because of blocked vessels in the legs and feet due to atherosclerosis.
Tissue Differences
Distribution occurs most rapidly into tissues with a greater number of blood vessels that allow high blood flow (such as the lungs, kidneys, liver, brain). Distribution occurs least rapidly in tissues with fewer numbers of blood vessels (such as fat), resulting in low blood flow. However, lipophilic drugs (i.e., drugs that dissolve in lipid environments) disproportionately distribute into adipose tissue in obese subjects.
The permeability of capillaries is tissue-dependent. Capillaries of the liver and kidney are porous, allowing for greater permeability. Distribution rates are relatively slower or nonexistent into the central nervous system because of the tight junction between capillary endothelial cells and the blood-brain barrier.
Protein-Binding
After a drug enters the bloodstream, a portion of it exists as free drug, dissolved in plasma water, but a portion of it becomes bound to proteins. This is important because only free and unbound drugs will pass from the bloodstream to tissues where drug-receptor interactions will occur, thus producing the first effects of a medication. The other portion of the drug that becomes “protein-bound” is inactive while it is bound. For many drugs, these bound forms can account for 95-98% of the total.[1]
Protein binding can also act as a reservoir as the drug is released slowly, causing a prolonged action. When considering drug distribution, it is important to consider both the amount of free drug that is readily available to tissues, as well as the protein binding that causes the drug to be released over time.
Albumin is one of the most important proteins in the blood. Albumin levels can be decreased by several factors such as malnutrition and liver disease. Therefore, clients with low albumin levels may experience differences in the desired actions of administered medication because of the consequence effect on protein-binding and distribution.
Competition for plasma binding can also impact the effects of drugs. For example, aspirin and warfarin are anticoagulants that compete for the same plasma protein-binding site. Administering both drugs at the same time will increase the amount of unbound drug, thereby increasing their effects and increasing the client’s risk for bleeding.[2]
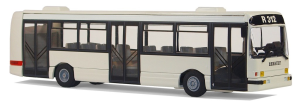
As an analogy of how protein binding affects the distribution of medications, consider passengers at a bus stop going to their destination. See Figure 1.4[3] for an image of a bus related to this analogy. Many passengers (i.e., drug molecules) want to take a ride on the bus. Everyone is eager to get to their destination (i.e., receptor sites) and tries to find a seat. Some passengers are stronger than others and take all the seats first (such as drug molecules with greater protein-binding ability). When there aren’t enough seats on the bus, some passengers are left at the bus stop and become “free” to move around or walk to their destination. In a similar way, “free” drug molecules that are not protein-bound circulate freely in the bloodstream. The “free” passengers in this analogy may go directly to their destination, or they may stop at other locations along the route. In a similar manner, “free” drug molecules produce the first intended or unintended effects in the body when they attach to receptors. Furthermore, similar to the passengers who had seats on the bus and then later got off at their destination, the medication molecules attached to proteins are eventually released and attach to the receptor sites.
Blood-Brain Barrier
Medications destined for the central nervous system (the brain and spinal cord) face an even larger hurdle than protein-binding; they must also pass through a nearly impenetrable barricade called the blood-brain barrier. This blockade is built from a tightly woven mesh of capillaries that protect the brain from potentially dangerous substances, such as poisons or viruses. Only certain medications made of lipids (fats) or those with a “carrier” can get through the blood-brain barrier.
Scientists have devised ways for medications to penetrate the blood-brain barrier. For example, the brand-named medication Sinemet® is a combination of two drugs: carbidopa and levadopa. Carbidopa is designed to carry the levadopa medication across the blood-brain barrier, where it enters the brain and is converted into dopamine to exert its effect on symptoms related to Parkinson’s disease.
Some medications inadvertently bypass the blood-brain barrier and impact an individual’s central nervous system function as a side effect. For example, diphenhydramine is an antihistamine used to decrease allergy symptoms. However, it can also cross the blood-brain barrier, depress the central nervous system, and cause the side effect of drowsiness. In the case of a person who has difficulty falling asleep, this drowsy side effect may be useful, but for a person trying to carry out daily activities, drowsiness can be problematic.
Placental Barrier
The placenta links mother and fetus, and the blood-placental barrier regulates transfer of molecules between maternal and fetal circulation to protect the fetus. Drug transporters are involved in transport of drugs through the placenta, affecting potential drug distribution to the fetus.[4] The placenta is known to be permeable to some medications, and furthermore, some drugs can cause significant harm to the fetus. However, many medications have not been specifically studied in pregnant clients and their effects on the fetus are unknown.
For this reason, it is always important to consider the potential effects of medication on the fetus if it is administered to a client who is pregnant or who may become pregnant. Nurses play a critical role in notifying the health care provider regarding potential safety concerns if medication can be distributed to the fetus. Nurses must always check a recent, evidence-based drug reference before administering medications to a client who is pregnant or may become pregnant. This imperative is implied in the remaining chapters.
Life Span Considerations
Neonate & Pediatric
Fat content in infants and children is decreased because of greater total body water. Additionally, protein-binding capacity is decreased, and the developing blood-brain barrier allows more drugs to enter the central nervous system.[5]
Older Adult
At the same body mass index, older adults, on average, tend to have more body fat than younger adults. This increased body fat can result in a longer duration of action for many medications that accumulate in fatty tissues. Serum albumin also decreases, resulting in more active free drug circulating within the body. For these reasons related to distribution, many older adult clients require lower dosages of medication.[6]
Media Attributions
- Renault Type r321 Service Bus is licensed under a CC0 (Creative Commons Zero) license
- This work is a derivative of StatPearls by Onetto and Sharif and is licensed under CC BY 4.0 ↵
- This work is a derivative of StatPearls by Onetto and Sharif and is licensed under CC BY 4.0 ↵
- “Renault Type r321 Service Bus” by Emslichter is licensed under CC0 1.0 ↵
- Liu, L., & Liu, X. (2019). Contributions of drug transporters to blood-placental barrier. Advances in Experimental Medicine and Biology, 1141, 505–548. https://doi.org/10.1007/978-981-13-7647-4_11 ↵
- Fernandez, E., Perez, R., Hernandez, A., Tejada, P., Arteta, M., & Ramos, J. T. (2011). Factors and mechanisms for pharmacokinetic differences between pediatric population and adults. Pharmaceutics, 3(1), 53–72. https://doi.org/10.3390/pharmaceutics3010053 ↵
- Fernandez, E., Perez, R., Hernandez, A., Tejada, P., Arteta, M., & Ramos, J. T. (2011). Factors and mechanisms for pharmacokinetic differences between pediatric population and adults. Pharmaceutics, 3(1), 53–72. https://doi.org/10.3390/pharmaceutics3010053 ↵
You have been working for six months on a medical telemetry floor and notice a change in your colleague Allie. Allie has been showing up to work late for her shifts and recently made a medication error when drawing up insulin. She mentions to you in the break room that she is having trouble sleeping and has been struggling with migraine headaches. Her husband was recently laid off from his position with a local manufacturing company, so Allie has been volunteering for extra shifts and overtime. Allie is typically good-natured and has a great sense of humor, but lately she has been short-tempered and irritable. As her friend and colleague, you want to see if there is anything that you can do to help
Reflective Questions
- Using your own words, how would you address your concerns with Allie?
- What strategies and assistance could you offer her?
As Allie’s colleague, you note many signs of harmful stress beginning to manifest in Allie’s physical, mental, and behavioral health. One of the first actions that you could take would be to invite Allie to discuss what you have noticed lately. Allie may not even realize that she has been exhibiting signs of ineffective coping and harmful stress. Opening the opportunity to discuss what you have seen and demonstrating that you acknowledge that she is in distress are important steps. Additionally, it is important to discuss with Allie what other resources are available to her. She may wish to reach out to the organization’s employee assistance program for assistance, or there may be other organizational resources available to her. Allie should also be encouraged to reach out to her manager to discuss the challenges that she is currently experiencing. This is an important part of mobilizing the resources around her so that she can continue as a successful employee within the organization.
Test your knowledge using this NCLEX Next Generation-style Case Study. You may reset and resubmit your answers to this question an unlimited number of times.[1]